3.2 The Role of Zirconium in Sm(Co, Cu, Fe, Zr)₇.₄ Permanent Magnetic Alloys: Insights from Electron Microscopye
This section is about directly observing precipitation and development of zirconium enriched long lamellar phase (or called Z phase) by 1000kV HVEM and combining with magnetism measurement to research coercivity mechanism of this alloy; and making use of Mössbauer effect to study impact of zirconium on the occupation status of each atoms crystal site and its relationship with coercivity.
Specimen Preparation and Experimental Methods for Studying Sm(Co, Cu, Fe, Zr)₇.₄
Specimen preparation for electronic microscope: Specimen components in experiment: 26.01%Sm, 51.38%Co, 4.36%Cu, 15.19%Fe, 2.99%Zr; solid solution treatment for 0.5h at 1180°C and then quenching to room temperature. The specimen was sliced to lamellae of the thickness of 0.25mm along the direction parallel to \(c\) axis. Then was electrolysis thinned by 20% perchloric acid and 80% glacial acetic acid to almost perforation. Afterwards the specimen was thinned and cleaned at last on ion thinning device.
Experimental method: The observation of filmy specimen was carried out using JEM - 1000 HVEM with operating voltage 1000kV, output voltage 333V, current 6.2A, vacuum \(3.32×10^{-4}\)Pa at 9.3×10-4Pa after adding liquid nitrogen, and ion beam 10μA. In experiment first specimen into JEM - 1000 side insertion heating experimental dais, and then carried out heating from room temperature to 850°C and held under electronic microscope.
Mössbauer analysis was carried out on constant acceleration Mössbauer Spectrometer, the specimen was powered. The experiment was proceeded at room temperature, radioactive source was \(^{57}Co(Rh)\) and the intensity is about Zomci, took count of was above \(2.5×10^{5}\), velocity demarcate adopted c-Fe standard spectra.
Investigating the Function of Zirconium in Sm(Co, Cu, Fe, Zr)₇.₄ Magnets
The in situ and dynamic observation using electronic microscope: At room temperature alloys appeared as single - phase status without precipitated phase in crystal granule except crystal boundaries and a small quantity of defects. When temperature rose to 460°C the cellular texture appeared on base phase. When temperature rose of 780°C the lamellar phase being perpendicular to \(c\) axis appeared on base of original cellular texture (Fig. 3.11). It can be seen from Fig. 3.11 that
this lamellar phase being perpendicular to c axis appeared in only part of whole visual field. At 780°C this lamellar phase being perpendicular to c axis appeared at most of all the visual field and at 790°C the lamellar phase being perpendicular to c axis appeared on base of original cellular texture. Along with continual rising of temperature the lamellar phase was increased gradually. Fig. 3.14 shows the long lamellar phase grown in the in situ at 810°C, Fig. 3.16 shows the lamellar phase grown in the in situ at 830°C and Fig. 3.17 shows the lamellar phase at 840°C and holding for 0.5h. It can be seen from Fig. 3.17 that the lamellar phase ran through the whole visual field.
The Mössbauer Effect experiment: According to sub - spectral crystal site of separation, spectrum was fitted and determined basis on analysis of \(RE_2Co_{17}\) and \(RECo_5\) crystal structures, \(RE_2Fe_{17}\) Mössbauer effect experiment, NMR (Nuclear Magnetic Resonance) experimental result of \(RE_2Co_{17}\) and \(RECo_5\). Crystal site marker, crystal sites \(Co_1\), \(Co_2\), \(Co_3\) and \(Co_4\) corresponds to 18g, 18h, 6c and 9d in \(RE_2Co_{17}\) diamond structure and crystal site 12j, 12k, 4f and 6g in \(RE_2Co_{17}\) hexagonal structure.
Average hyperfine field \(H_{hf}\) was obtained by weighted sum of each crystal site \(H_{hf}(i)\) and area percentage \(A_i\), that is:
The 2:17 phase sub - spectral area increased from 75.1% in 0Zr specimen to 78.6% in specimen of 2.4Zr. It can be seen that iron atoms entered into the 2:17 phase from 1:5 phase by adding zirconium. The average hyperfine field \(H_{hf}\) of the 2:17 phase increased from 298.4kOe in specimen of 0Zr to 301.0kOe in specimen of 2.4Zr, and the average hyperfine field \(H_{hf}\) of the 1:5 phase decreases from 274.4kOe in specimen of 0Zr to 270.2kOe in specimen of 2.4Zr. These indicated that zirconium could make nonmagnetic atoms, zirconium and copper, decomposed from the 2:17 phase to the 1:5 phase because exchange interaction of Fe - Fe in the 1:5 phase (or the 2:17 phase) reduced (or increased) magnetic moments of iron and thus reduced (or increased) hyperfine field at \(^{57}Fe\) nuclei. This is consistent with the result of energy spectrum analysis that zirconium enriched phase appeared in specimen of 2.4Zr. According to opinion of Panl, coercivity of alloy was related to different in magnetic characters between two phases (magnetocrystalline anisotropy, saturation magnetization intensity and exchange interaction) as well as size and distribution of defects. Therefore, addition of zirconium increased nonmagnetic atoms in the 1:5 phase and reduced magnetism of the 1:5 phase, and made domain wall intensified and the coercivity enhanced.
Addition of zirconium can promote iron atoms enter into \(Co_3\) crystal site from \(Co_1\) crystal site. In the 2:17 phase \(Co_1\) crystal site has strong axial anisotropy, \(Co_3\) crystal site is of strong plane anisotropy, and \(Co_2\) and \(Co_4\) crystal sites only have weak plane anisotropy. And that iron atom replacing cobalt will weaken anisotropy. Therefore increase in number iron atoms occupying \(Co_3\) crystal sites will weaken plane anisotropy of these crystal sites and decrease in number iron atoms occupying \(Co_3\) crystal sites will enhance the axial anisotropy of these crystal sites. As the reduction it is to enhance the single axial anisotropy in these crystal sites. The result is increasing alloy’s single axial anisotropy and so that to be avail of enhancement of coercivity (Strnat, 1983; Pan, Xiao, 1989). The process proved that zirconium played a key role in improving alloy coercivity. It is advantaged also to enhance quadrate degree in demagnetization curve. Adding zirconium can increase solubility of samarium in the alloy that the solubility of Samarium in \(Sm_2(Co,Cu,Fe,M)_{17}\) is increased by 27%.
It can be seen from Fig. 3.30 that coercivity \(_{m}H_c\) of the 25.2Sm - Co - 6Cu - 15Fe - Zr alloy increased along with increasing of zirconium content, and the ratio of \(H_k/_{m}H_c\) increased with increasing of zirconium. \(H_k/_{m}H_c\) indicated the ridgy degree, or called quadrate degree of demagnetization curve. The quadrate degree has directly related to big and small of \(B_r\), \(H_c\) and \(H_k\) and also related to components and heat treatment process of the alloy. People always want to get demagnetization curve with good quadrate degree. In \(Sm(Co,Cu,Fe,M)_z\) alloy \(_{m}H_c\) was trebled to reach 2000kA/m with zirconium content of 2% (at.) in compared with content of zirconium (at.) 0% (in condition \(TbCu_7\) structure, and single - phase region 11.5% - 12.5%(at.)Sm).
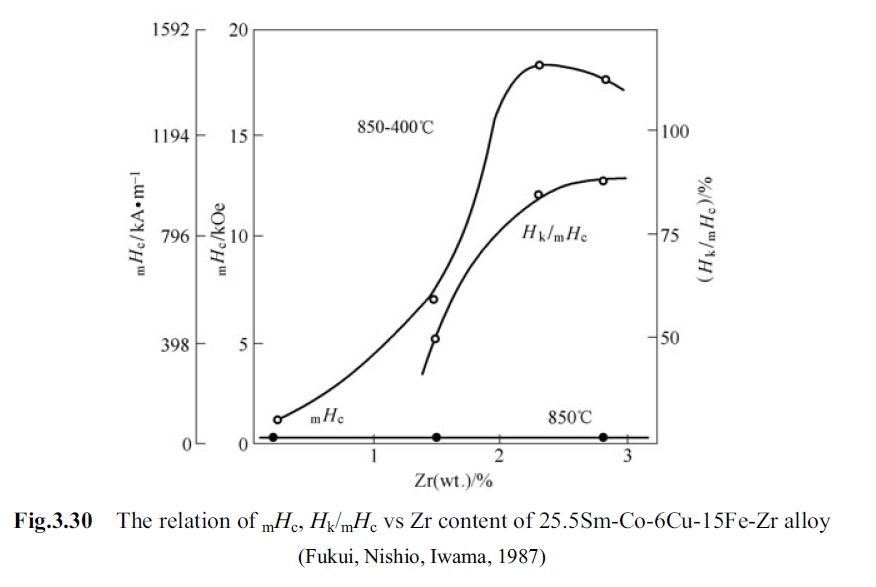
Adding zirconium into zirconium enriched 2:17 phase made 2:17 phase extending to cobalt enriched and samarium enriched sides. Then solubility of samarium in the 2:17 phase was enhanced to 13% (at.) from 9.5% (at.) at 1370°C and thus changed structure of high temperature phase and fully exerted effect of zirconium. For the 2:17 alloys containing zirconium multistage aging were adopted (as shown in Fig. 3.20). When content of zirconium is less than 1.5% isothermal aging can be controlled for 30min to 40min. When \(Zr > 2.0\%\) (at.) isothermal aging is controlled in 9 - 35h. Respective aging temperatures and holding times are at 700 - 600°C for 1h, 500°C for 2h, and 400°C for 4h. For alloys with high content of zirconium generally cool to 400°C then holding for 3h with the cooling speed of 0.3% - 1.5%/°C. For permanent magnet with \(Zr\geq3\%\) (wt.) isothermal aging at 850°C makes against enhancement coercivity. The alloy no matter with a low-zirconium or a high-zirconium have to process classifying aging after isothermal aging at 820 - 850°C and the cooling speed is 0.3 - 1.2°C/min in order to obtain high performance permanent magnetic alloy.
From above analysis, we can know that zirconium plays a key role for enhancement of coercivity of the \(Sm(Co, Cu, Fe, M)_{7.4}\) alloy.
Conclusions: The Impact of Zirconium on the Structure and Magnetism of Sm(Co, Cu, Fe, Zr)₇.₄
The following conclusions are derived based on above researches:
- Dynamic observation on \(Sm(Co, Cu, Fe, Zr)_{7.4}\) using 1000kV TEM proves, appearance temperature of zirconium enriched long lamellar phase is 790°C. Holding at 840°C for 0.5h this texture runs through whole view field. This long lamellar structure is not the direct reason to cause produce high coercivity.
- According to the Mössbauer research addition of zirconium can promote iron enter into the 2:17 phase from the 1:5 phase. Meanwhile nonmagnetic ions, copper, zirconium, etc. enter into 1:5 phase from 2:17 phase. These increased the difference in chemical composition and magnetic character between two phases. Addition of zirconium promote iron atoms in the 2:17 phase enter into \(Co_3\) crystal sites from \(Co_1\) crystal sites, consequently enhance uniaxial anisotropy of the alloy. And these two points both are favorable to enhance coercivity of the alloy.
- Zirconium in \(Sm(Co, Cu, Fe, Zr)_{7.4}\) alloy acts enhancing coercivity, increasing solubility of samarium in the alloy, and improving quadrate degree of demagnetization curve.