4.13 Stability of Rare Earth Permanent Magnetic Alloys: Temperature, Time, and Chemical Resistance
The stability of rare earth magnets include temperature stabil-ity, time stability, mechanism stability, magnetic stability, radiation stability, chemical stability, etc. These stabilities are as important as the magnetic proper-ties of rare earth permanent magnetic alloy.
Thermal Stability of Rare Earth Permanent Magnetic Alloys
Change of outside temperature (environment temperature) causes variety in mag-netic performance of permanent magnetic alloy. The magnetic capability of the rare earth permanent magnet will not degrade by using at or below room tempera-ture. When used at high temperature the magnetic capability will degrade or ap-pear irreversible loss. \(Nd_{14}Fe_{77}B_{8}\) is used stably at \(80^{\circ}C\) or below. However, the requirement of permanent magnet for aviation and space - flight must be working stably at an environment of \(200^{\circ}C\). High capacity or appear irreversible loss. \(Nd_{14}Fe_{77}B_{8}\) is used stably at \(80^{\circ}C\) or below. However, the requirement of permanent magnet for aviation and space - flight must be working stably at an environment of \(200^{\circ}C\). High capacity rare earth permanent magnet is used in magnetic force damper, magnetic force sensor and power apparatus of high rotation speed for spacecraft works in mediums of liquid hydrogen and liquid oxygen. Accordingly, inspection at normal temperature and power apparatus of high rotation speed for spacecraft works in mediums of liquid hydrogen and liquid oxygen. Accordingly, inspection at normal temperature will not satisfy the requirements because it works at lower temperature. Therefore, requirement for temperature stability has to be necessary for the rare earth perma-nent magnetic alloy (Pan, Ma, Li, 1993; Pan, Ping, Liu, et al, 2003; Pan, Zhao, Ma, 1988; Pan, Li, Li, et al, 1989; Pan, Li, 2000; Pan, Chen, Liu, et al, 1994; Tang, Feng, Luo, Pan, 1994).
The Fig. 4.74 shows that remanence \(B\) in open circuit of permanent magnetic materials vary with the change of temperature. The temperature stability of per-manent magnetic alloy can be described use the fore parameters (Zhou, Dong, 1999; Pan, 2011):
\[ h_{T}=\frac{B(T)-B(T_{0})}{B(T_{0})}\times100\%=\frac{\Delta B_{r}}{B(T_{0})}\times100\% \quad (4.9) \] \[ h_{ir}=\frac{B'(T_{0})-B(T_{0})}{B(T_{0})}\times100\% \quad (4.10) \] \[ h_{rev}=\frac{B(T)-B'(T_{0})}{B'(T_{0})}\times100\% \quad (4.11) \] \[ \alpha=\frac{B(T_{1})-B'(T_{0})}{B'(T_{0})dT}\times100\% \quad (4.12) \] where \(h_{T}\) is total loss of remanence \(B\) in open circuit (flux in open circuit); \(h_{ir}\) is irreversible loss of flux \(B\) in open circuit; \(h_{rev}\) is reversible loss of flux \(B\) in open circuit; \(\alpha\) is reversible temperature coefficient at temperature \(T_{1}\), \(\%\)/\(^{\circ}C\).
Aging treatment is easily to be neglected in manufacturing process of permanent magnetic materials. The so - called aging treatment is to improve the homogeneity of permanent magnetic alloy by heating at a certain temperature. The normal ag-ing treatment method is to hold in atmosphere for 1 - 3 hours at \(80 - 200^{\circ}C\). The permanent magnetic alloy through aging treatment is to hold in atmosphere for 1 - 3 hours at \(80 - 200^{\circ}C\). The permanent magnetic alloy through aging treatment eliminates the factors of domain structure and instable in the alloy.
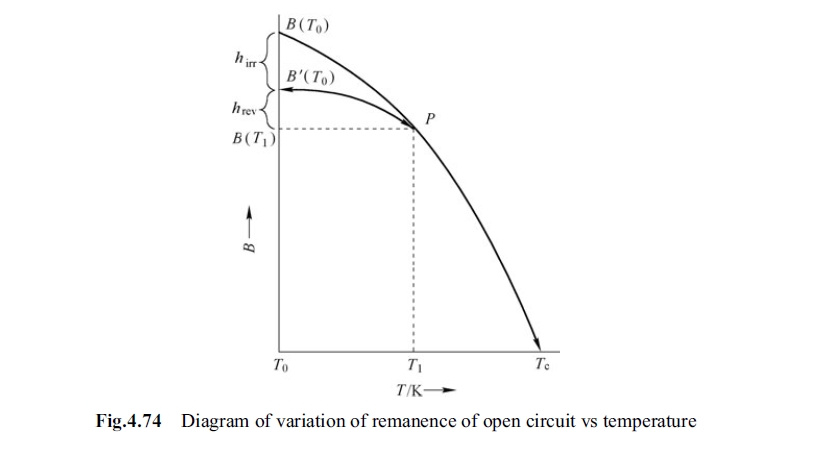
Stability on temperature for alloys of Sm (Co, Cu, Fe, Zr)₂ and RECo₅
In order to review the stability on temperature of \(SmCo_{5}\) and \(Sm (Co, Cu, Fe, Zr)_{7.4}\) alloys we measured these two alloys at high and low temperature of 1.5 K and 523 K.
Procedures of measurement are as follows:
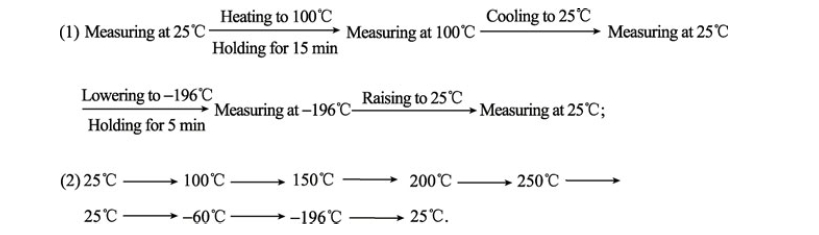
The conclusion is derived after measurement as follows.
-
Magnetic performance of permanent alloys of \(SmCo_{5}\) and \(Sm (Co, Cu, Fe, Zr)_{7.4}\) degrade with raising temperature, upgrade with lowering temperature, and have good reversion ability.
-
\(SmCo_{5}\) permanent magnetic alloy of 2:17 type Sm - Co has very good reversion ability at temperature range from \(-196^{\circ}C\) to \(200^{\circ}C\).
-
The \(SmCo_{5}\) permanent magnetic alloy does not have magnetic irreversible loss at low temperature.
-
The stability regularity is derived by measuring several specimens of the above mentioned first and second generation rare earth permanent magnetic alloy at 1.5 - 523 K:
-
The specimen with a high intrinsic coercivity has good thermal stability;
-
The coercivity of \(SmCo_{5}\) permanent magnetic alloy degrade to the lowest value at \(750^{\circ}C\) by annealing from room temperature to \(1000^{\circ}C\);
-
The \(SmCo_{5}\) permanent magnetic alloy appears the most severe irreversible magnetic loss at \(750^{\circ}C\) by annealing from room temperature to \(1000^{\circ}C\);
-
Any alloy with a high intrinsic coercivity will have, if occurs, a smaller ir-reversible magnetic loss as well.
After being placed at \(200 - 300^{\circ}C\) for a long term the irreversible flux loss is zero for \(Sm (Co, Cu, Fe, Zr)_{7.4}\) alloy; if being placed at \(350^{\circ}C\) the irreversible loss will arisen; after aging treatment at \(400^{\circ}C\) the irreversible loss accounts for 70% in a part of specimens and the reversible loss account for 30% (Zhou, Dong, 1999).
Thermal stability of NdFeB permanent magnetic alloy
The third generation of the rare earth permanent alloy, NdFeB, have a big im-provement in comparison with the first and second generations. Nevertheless, it have a low Curie temperature of \(312^{\circ}C\) only (while the Curie temperature of \(SmCo_{5}\) and 2:17 type Sm - Co alloys is above \(700^{\circ}C\)), the magnetic anisotropy field \(H_{A}\) of its magnetic phase (\(Nd_{2}Fe_{14}B\)) is not very high as well, and that it is sensitive to temperature so that the remanence and coercivity of NdFeB magnet will degrade after being heated. The irreversible temperature coefficient of NdFeB is rather big (\(\alpha_{Br}=0.12\%/^{\circ}C\)). Therefore, improving Curie temperature, lowering temperature coefficient and improving thermal stability of NdFeB mag-net is a study subject of theoretic and practical significance (Pan, Ping, Liu, et al, 2003; Pan, Zhao, Ma, 1988; Yang, Yang, 1993; Xu, Ping, Li, Ma, Pan, 1986; Liu, Pan, Luo, etal, 1991).
Author has conducted a series studies to improve thermal stability of NdFeB al-loy. The main way is alloying method. By adding dysprosium, niobium, cobalt, aluminum and gallium, in individual or combinatorial, into NdFeB alloy has got a favorable result in improving its thermal stability (Pan, Ma, Li, 1993; Pan, Ping, Liu, et al, 2003; Pan, Zhao, Ma, 1988; Pan, Li, Li, et al, 1989; Pan, Li, 2000; Pan, Chen, Liu, et al, 1994; Tang, Feng, Luo, Pan, 1994; Liu, Pan, Luo, 1991; Zhao, Geng, 1991; Wang, Pan, et al, 1999).
The experimental method is: use neodymium, dysprosium, iron, niobium, co-balt, aluminum, gallium and boron materials with purity above 99.5%, prepare with a certain proportion after properly purifying, melt in a medium frequency induction furnace or non - self - consuming electric arc furnace. Then comminute the ingot into powder of 3 - 4 μm under protection of medium, shape up in a magnetic field abled 1.2 T, sinter in a high temperature furnace of 1100 - 1200°C, pass through aging at \(900^{\circ}C\) and \(500 - 630^{\circ}C\) respectively, and cool to room temperature. Magnetize the specimen which is in thermo - demagnetization status and measure magnetism of the specimen (Pan, Ping, Liu, et al, 2003).
A. Adding dysprosium and adding dysprosium and aluminum together into NdFeB alloy
Addition of dysprosium proceeded from cognition as follows: in point of view from main phase of NdFeB alloy, i.e., NdFeB magnetic phase using dysprosium to substitute neodymium will obtain a much higher anisotropy field (\(H_{A}\)), as shown in the Table 4.22.
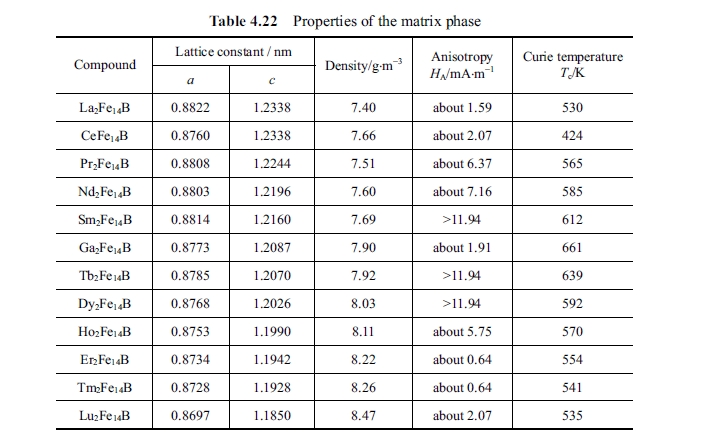
In addition, adding dysprosium into NdFeB ternary alloy can improve coerciv-ity of magnet.
Confect the material referring \((Nd_{1 - x}Dy_{x})_{16}Fe_{77}B_{7}\) formula and suppose \(x = 0.005, 0.010, 0.020, 0.030, 0.040, 0.050, 0.070, 0.090\), and obtained magnetism of alloys as shown in Table 4.23.
It can be seen from the Table 4.23 that the remanence induction strength \(B_{r}\) and maximum product of magnetic energy \((BH)_{max}\) decline with increase of dyspro-sium content in \((Nd_{1 - x}Dy_{x})_{16}Fe_{77}B_{7}\) alloy, but the coercivity is improved. The thermo - stability is also mentioned and will be heightened with increase \(x\) (the Curie temperature has not significant change, within the scope of tolerated error). The ternary NdFeB alloy is appropriate to be used within \(80^{\circ}C\), that the environ-ment temperature exceeding \(80^{\circ}C\) will result in severe decline in magnetic capa-bility. While \(x = 0.3\) the above mentioned alloy can be used at an environment
temperature of \(100^{\circ}C\); when \(x = 0.5\) the alloy can be used at an environment tem-perature of \(120^{\circ}C\); when \(x = 0.9\) the alloy can be used at an environment tempera-ture of \(150^{\circ}C\) (because the irreversible loss is less than 3% when the specimen is toasted at relevant temperature).
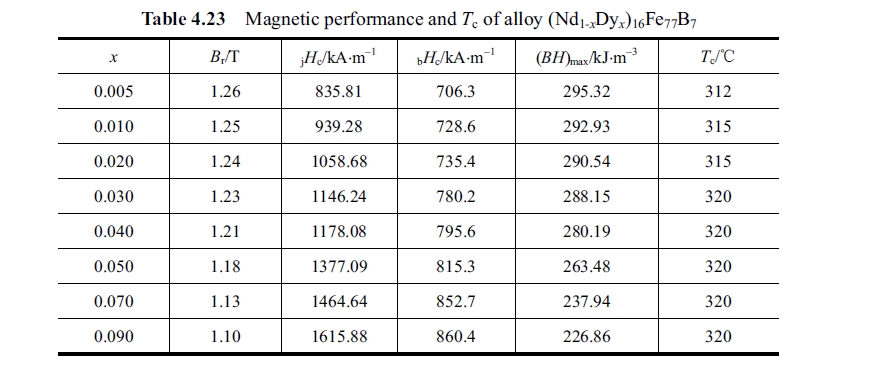
Previously experiments indicate that addition of aluminum into NdFeB alloy is able to improve the coercivity of the alloy. Thus combined addition of a little aluminum and dysprosium is able to improve the coercivity, and that to improve thermo - stability of the NdFeB alloy. As the result the alloy becomes a quinary alloy, it can be described precisely as \((Nd_{1 - x}Dy_{x})_{16}(Fe_{1 - y}Al_{y})_{77}B_{7}\). Among them selecting \(x = 0.020 - 0.040\), \(y = 0.01 - 0.03\) can obtain rather satisfied applicable magnet.
B. NdFeB alloy after adding niobium
Confect the material referring \((Nd_{1 - x}Nb_{x})_{16}Fe_{77}B_{7}\) formula and suppose \(x = 0.01, 0.02, 0.04, 0.08, 0.15\), and obtained magnetism of alloys as the alloys as shown in Table 4.24.
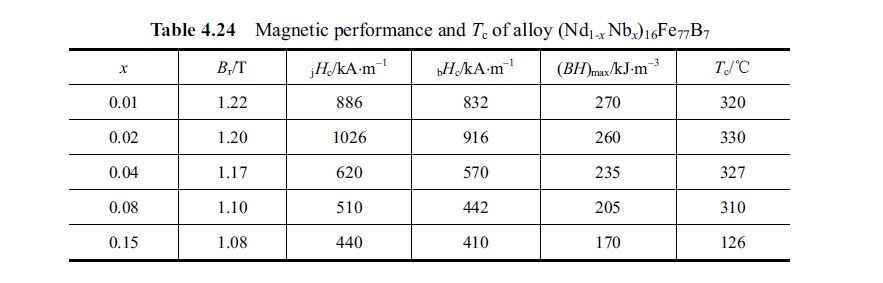
It can be seen that as the result the remanence induction strength and maximum product of magnetic energy \((BH)_{max}\) were declined monotonously by using a little niobium to substitute iron. And that the coercivity strength appears a peak value at \(x = 0.02\). This indicates that adding niobium can improve coercivity. Addition of niobium improves the square degree of demagnetization curve, referring to the curve. And addition of niobium is avail to increase dispersion and suppress growth of crystals. This point is very good for heightening coercivity of NdFeB and improving thermo - stability of magnet. In view of Curie temperature when \(x = 0.01\) the Curie temperature can be taken as unchanged. But as \(x = 0.08\) there are two ferromagnetic phase of \(T_{c1}=110^{\circ}C\) and \(T_{c2}=305^{\circ}C\) from thermomagnetic curve.
C. NdDyFeNb magnet by adding dysprosium and niobium together
Addition of dysprosium and niobium in same time has advantage over individual use of them. As a result the improvement of coercivity and a nicer quadrate degree in demagnetization curve of the maximum product of magnetic energy also be heightened correspondingly. Thus results in a good thermo - stability of the magnet.
D. NdFeB magnet with cobalt
The purpose of addition of cobalt is to aim at the low Curie temperature. There are many research reports about influence of cobalt on main phase of \(Nd_{2}Fe_{14}B\). Cobalt belongs to the transition family. The main phase becomes \(Nd_{2}(Fe_{1 - x}Co_{x})_{14}B\) after adding cobalt. Contribution of elements from transition family to intermetallic compound follows Slater - Pauling relation, i.e., \(\mu_{m}=2.6 - x\). Magnetic property and \(T_{c}\) of \((Nd_{1 - x}Fe_{1 - x}Co_{x})_{16}Fe_{77}B_{7}\) is shown in Table 4.1.
It can be seen from Table 4.1 that \(B_{r}\) and \((BH)_{max}\) will decline monotonously by using cobalt to substitute part of iron. For \(Nd_{2}(Fe_{1 - x}Co_{x})_{14}B\) the cobalt raised each \(8\%\) the Curie temperature can be heightened \(50^{\circ}C\) (for the main phase of \(Nd_{2}Fe_{14}B\) cobalt substituting each \(1\%\) of Fe can heighten Curie temperature \(10^{\circ}C\) for 2 - 14 - 1 main phase ). The higher cobalt content may not lower irreversible magnetic loss. But increasing cobalt may lower irreversible temperature coeffi-cient of remanence in open circuit (when \(x = 0.1\), \(\alpha_{Br}=-0.07\%/^{\circ}C\)). The reason is mainly due to the formation of \(Nd(Co, Fe)_{2}\) magnetically soft phase. The \(Nd(Co, Fe)_{2}\) may become nucleating center in magnetization process. However, combined addition of cobalt, dysprosium, niobium and gallium to substitute part of iron ob-tained very satisfied result and so as to heightened thermo - stability of NdFeB magnet.
E. NdFeB magnet with cobalt, dysprosium, niobium and gallium
Magnetic performance of NdFeB magnet with cobalt, dysprosium, niobium and gallium, being added together, is listed in Table 4.3 and Table 4.25.
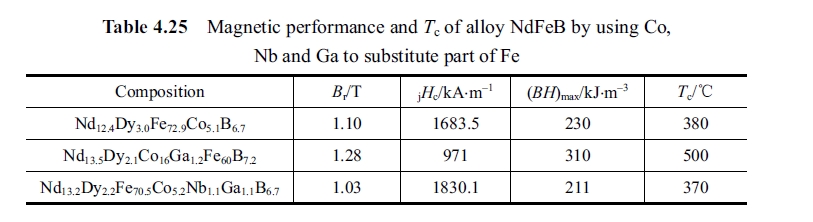
Time Stability of Rare Earth Magnets: Aging and Performance Retention
Placed the permanent magnet at a long term at a certain temperature and meas-ured the variation of magnetic property with change of time. For \(SmCo_{5}\) alloy being placed for 1000 hours after heat treatment at \(200 - 250^{\circ}C\) for \(1 - 2\) hours the decline of remained magnetic induction was less than 1%. Generally speaking without this aging experiment the remained magnetic induction would decline obviously at \(200 - 250^{\circ}C\) for 1 hour and afterwards the variety tends to be smoothly.
Temperature of time stability is among \(25 - 250^{\circ}C\) and the measurement can be planned at the temperature in accordance with the requirement.
Chemical Stability of Rare Earth Permanent Magnetic Alloysy
The permanent magnet works in the environment of a certain acidic or alkaline of chemical workshop or laboratory. That requires the permanent magnet being pro-vided with corrosion resistant property. Place the specimen into corrosive medium and then measure its coercive speed. The \(RECo_{5}\) permanent magnetic alloys are the best rare earth permanent magnetic alloys in chemical stability in comparison with the 2:17 Sm - Co type and NdFeB alloys. However, among \(RECo_{5}\) alloys it is the best when RE is Sm i.e., \(SmCo_{5}\). If RE being Pr or \((CeMM)Co_{5}\) they are not as so good as \(SmCo_{5}\) in chemical stability. 2:17 type Sm - Co permanent magnet alloy is the best in oxidation resistance, while rare earth ferromagnetic alloys are not as good as rare earth cobalt base alloy in chemical stability. NdFeB alloy uses surface coating for oxidation resistance, normally adopting nickel or zinc coating to protect permanent magnetic alloy.
Conclusions: Long-Term Performance and Stability of Rare Earth Magnets
-
Addition of appropriate cobalt content into NdFeB ternary alloy can height-ens Curie temperature of the alloy from \(312^{\circ}C\) to \(500^{\circ}C\). However, addition cobalt alone can heighten the Curie temperature and lower the irreversible temperature coefficient, but can not reduce the irreversible loss. To improve the thermal stability it is more effective to add other elements together with cobalt.
-
Thermal stability of NdFeB alloy can be improved greatly through alloying method which is a brief and effective approach for the purpose. But it is very strict for adding what kind of element and how amounts of the elements. Addition of appropriate amounts of dysprosium, niobium, gallium and/or alumi-num can uniformly heighten the coercivity of the alloy. And through heightening coercivity is an effective way to improve the thermo - stability of the alloy.
-
Using a little dysprosium to substitute neodymium, using a little niobium, aluminum, cobalt and gallium to substitute part of iron and adjusting a propor-tional relationship of amounts can obtain an applicable magnet with high coerciv-ity and high maximum product of magnetic energy. This magnet will have the thermal stability about \(70 - 100^{\circ}C\) higher than that of ternary NdFeB alloy.
-
Any rare earth permanent magnetic alloy which possesses a high coercivity will have a better thermal stability and smaller irreversible loss. Heightening co-ercivity is an effectual approach to improve stability of the rare earth permanent magnetic alloy.
-
\(SmCo_{5}\) permanent magnetic alloy has a very good reversion property at the temperature from \(-196^{\circ}C\) to \(200^{\circ}C\). It has no irreversible loss at low temperature. The irreversible loss will reach the maximum by annealing at \(750^{\circ}C\). And that 2:17 type Sm - Co alloy is the best in oxidation resistance.
-
The rare earth cobalt base permanent magnetic alloy has the chemical stabil-ity, time stability and thermal stability better than those of the rare earth ferrous base permanent magnetic alloy. Aging experiment avails stability of mother alloy.