5. Developments and Future Prospects of Rare Earth Permanent Magnet Alloys
The Rare earth magnet alloy - magnet alloys are the broadly used foundational func-tional materials. Rare earth permanent - magnet alloys have achieved great pro-gress in scientific research, manufacturing and application in recent decades, and their applications have pervaded into every region of national economy. Rare earth permanent - magnet alloy has become important material basis of new tech-nology.
China is not only rich in the storage capacity of rare earth material, but also af-fluent in output. Nowadays, improving the performance of rare earth permanent magnetic alloy and searching for new generation with outstanding performance has become one of focuses being paid most attention to this field.
The development of nanometer science and technology brings new challenges and opportunities for the rare earth permanent magnetic industry. Nanometer bril-liant exchange coupling is the permanent magnetic material which is one of the material with brightest future and the development of this material and correlation technique certainly promote the technical progress of rare earth permanent mag-netic alloys and upgrade performances of traditional products, and thus it will result in development of high and new technology products for rare earth perma-nent materials.
Two - phase composite nanocrystalline rare earth permanent magnet is a kind of promising magnetic material. Their theoretical maximum magnetic energy prod-uct can reach to \(993.75kJ/m^{3}\), which as twice as that of NdFeB magnets. And the low rare earth content gives them great advance in cost efficiency. Additionally, the better corrosion resistance and high Curie temperature broaden their appli-cation area. The development of this material will gear up the development of rare earth permanent magnets and the performance of traditional magnets.
Global Developments in Rare Earth Permanent Magnet Alloys
Rare earth metals, which exist in rare earth permanent magnetic alloys as alloy elements, transform into 3d transition intermetallic compounds together with 3d transition metals. Among them rare earth cobalt base and rare earth iron base in-termetallic compounds have been successfully applied in electric machine, in-strument, petrochemical industry, automobile, automation and computer area.
Rare earth cobalt based permanent magnetic alloy started in 1960s. Early in 1959, E. A. Nesbitt, et al (Wesbitt, et al, 1959) and in 1960 W. M. Hubbard, et al found \(GdCo_{5}\) alloy (Hubbard, Adams, Gilfrich, 1960) successively. In 1966, G. Hoffer, et al. found that \(K_{1}=5.7\times10^{6}J/m^{3}\), \(\mu_{0}M_{s}=1.06\ T\) (Hoffer, Strat, 1966) for \(YCo_{5}\). In 1967, K. J. Strat, et al. firstly made out permanent magnetic alloy \(YCo_{5}\) using powder metallurgy technique, but its performance was very low, \((BH)_{max}=9.6\ kJ/m^{3}\); in succession they synthesized permanent magnetic alloy \(SmCo_{5}\) using Sm substituted for Y by element substitution method, and the magnetic character-istics were as follows: \(B_{s}=0.51\ T\), \(_sH_{c}=254.7\ kA/m\) and \((BH)_{max}=40.6\ kJ/m^{3}\) (Strat, Hoffer, Olson, et al, 1967). In 1968, K. H. Bushow, et al. prepared perma-nent magnetic material using powder metallurgical technique to enhancing alloy density which created new record of product of magnetic energy at that time: \((BH)_{max}=147.3\ kJ/m^{3}\) and the intrinsic coercivity \(_mH_{c}=1257\ kA/m\) (Bushow, et al, 1968). The technique of permanent magnetic alloy \(SmCo_{5}\) was come to perfection during the year 1969 to 1972: M. G. Benz, et al. improved the performance of the alloy using the method of liquid - phase sintering in 1970 (Benz, Martin, 1970). In 1972, R. J. Chaless, et al. used reduce - diffusion (\(R/D\)) method prepared permanent magnet (\(Pr_{0.5}Sm_{0.5})Co_{5}\) with \((BH)_{max}=207\ kJ/m^{3}\) (Chaless, et al, 1972). In 1973, A. C. Ермоленко, et al. synthesized the single crystal of \(SmCo_{5}\) creating new record in high magnetic characters \((BH)_{max}=254.7\ J/m\) (32 MG・Oe) (Ермоленко, 1973). As the representative of rare generation rare earth permanent magnetic alloy \(SmCo_{5}\) has main performance as follows:
To acquire the magnetic performance as shown in the Table 5.1 the alloying com-position must be calculated as per the compound molecular formula. The compo-sition is samarium 36% - 37% and cobalt 63% - 64% in mass fraction. High tem-perature sintering is the key factor to form high performance alloy; the heat - treatment system are holding for more than 1 h at \(1120^{\circ}C\), cooled to \(900^{\circ}C\) in speed of \(0.6 - 0.9^{\circ}C/min\) and holding for 3 - 5 h then quenching to room temperature. \(SmCo_{5}\) has the biggest magnetic anisotropy and is much easier than other rare earth permanent magnetic alloys to gain high coercivity. The main processes of producing \(SmCo_{5}\) using sintering process (alloy melting process) are alloy melting \(\to\) milling \(\to\) shaping in magnetic field \(\to\) aging \(\to\) processing \(\to\) magnetizing. To prevent oxidization melting, milling and sintering should be carried out under the protection of argon atmosphere.
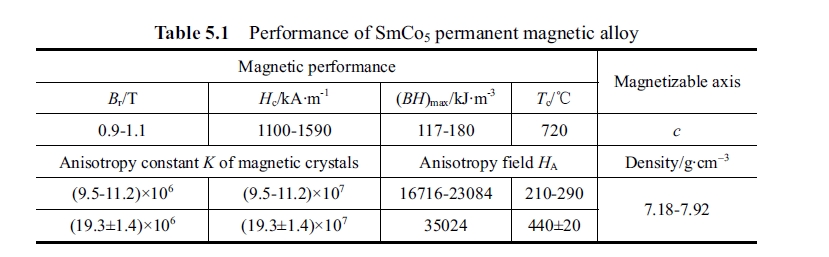
The mechanism of coercivity of \(SmCo_{5}\) belongs to nucleation mechanism, that is to say, the coercivity of the alloy is controlled by its nucleation field and the formation of reversal magnetization domain should be in upfield.
The secondary generation rare earth permanent magnetic alloys formally came into being in 1977, when T. Ojima, et al. successfully made the rare earth perma-nent magnet of the composition of \(Sm(Co, Cu, Fe, Zr)_{7.2}\) using powder metallur-gical technique. That rare earth permanent magnet created the highest record in product of magnetic - energy at that time with: \((BH)_{max}=238.8\ J/m^{3}\) (30MGs·Oe). Its development process spent ten years. At first in 1968, E. A. Nesbbit, et al. used cupper to substitute part of cobalt prepared intermetallic compound of \(Sm(Co,Cu)_{5}\) and obtained the permanent magnetic alloy with \((BH)_{max}=31.8 - 55.7\ J/m^{3}\) (Nesbbit, Willens, Sherwood, et al, 1968). In 1974 A. J. Perty, et al. and in 1976 A. Menth, et al. researched \(Sm((Co + Fe)_{1 - x - y}Cu_{x}M_{y})_{5 - 8.5}\) alloy and prepared practical applicable rare earth permanent magnetic alloy. The performances of the second generation rare earth permanent magnetic alloy \(Sm(Co, Cu, Fe, Zr)_{z}\) (\(z = 7 - 8.5\)) are showed in Table 5.2.
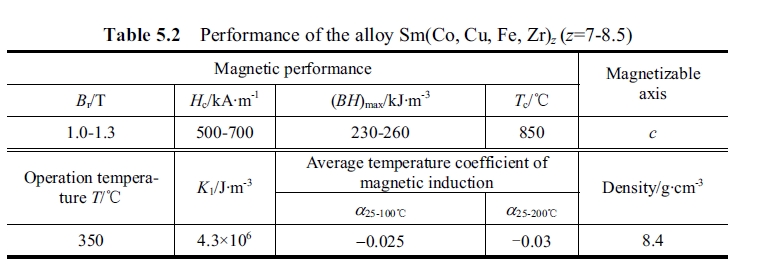
In order to acquire high performances shown in Table 5.2, a special heat treat-ment processes should be adopted, which include solid solution treatment and isothermal aging. The solid solution treatment is aim to acquire homogeneous monomial solid solution, and solid solution temperature is at \(1130 - 1170^{\circ}C\) while grade aging treatment at below \(850^{\circ}C\). After solution treatment and isothermal aging treatment at below \(850^{\circ}C\), the alloy is of exiguous cellular microtexture in the state of high coercivity. The diameter of cell is about 50 nm and cell wall thickness is about 5nm. When aging at \(830^{\circ}C\) for more than 20 h the cellular texture of the alloy becomes incomplete and part of it is destroyed. The fab-rication process of alloy is to put the prepared raw material as per nominal com-position into furnace for melting at the protection of argon atmosphere at first, sec-ondly quench melted alloy in water cooled mold, and the third alloy mill and then mold ingot in magnetic field; afterwards, process stage aging as per above men-tioned heat treatment system. Through multistage aging (precipation hard-ening, producing two stage decompound) the coercivity of the alloy can be im-proved in a large magnitude, the maximum magnetic energy product of the alloy can reach \(250\ kJ/m^{3}\) or above.
The magnetic anisotropy constant \(K_{1}\) and anisotropic field \(H_{A}\) of the secondary generation rare earth permanent magnetic alloys are both less than those of the first generation, and so is the coercivity. The coercivity of alloy depends on pin-ning field because the pinning field and initial magnetization curve enhance gradually along with increase of magnetic field and at last reaches saturation (the magnetic field must be bigger than the pinning field), its coercivity is determined by the structure of two phases, i.e., when magnetization and reverse magnetiza-tion of domain wall of \(Sm_{2}Co_{17}\) is pinned by \(SmCo_{5}\), which enhanced the coer-civity.
Space aeronautic and aviation fields requires that the rare earth permanent magnetic materials have good performance and thermal stability at \(400 - 500^{\circ}C\). The 2:17 Sm - Co permanent magnetic alloy becomes preferred material for the requirement. In 2000 EEC corporate, USA, produced Sm - Co magnet with the maximum magnetic energy product of \(79.6\ kJ/m^{3}\) and coercivity of \(95\ kA/m\) at \(500^{\circ}C\).
In China the Research Institute of Iron & Steel of Beijing used near fast solidi-fication technique to prepare permanent magnetic alloy with the maximum mag-netic energy product of \(79.89\ kJ/m^{3}\) and coercivity of \(95\ kA/m\) at \(500^{\circ}C\) by changing iron content to control the coercivity coefficient.
The Curie temperature and saturation magnetization intensity of the secondary generation rare earth permanent magnetic alloys are both less than those of the first generation; the second generation has a smaller reversible temperature coefficient and a better thermal stability as well, so it is fit for using at higher temperature.
The third generation rare earth permanent magnetic alloy was made by M. Sa-gawa, et al. of Sumitomo Special Metals Corporation of Japan in 1983 using pow-der metallurgic method. The alloy was the \(Nd_{15}Fe_{77}B_{8}\) permanent magnetic alloy with \((BH)_{max}=286.6\ kJ/m^{3}\) (36MGs·Oe), which created the highest record in magnetic - energy product at that time (Sagawa, Fujimura, Togawa, et al, 1984). It was based on the researches and studies of many scholars and scientists. According to early researches people found that \(REFe_{2}\) and other rare earth series have higher coercivity at low temperature and hard magnetic properties of amorphous material \(REFe_{2}\) was improved during crystallization. During 1972 to 1973, A. E. Clark and made compound TbFe₂ into amorphous state at room temperature and annealed, as a result its coercivity improved in big magnitude to \(_mH_{c}=273\ kA/m\) (3.4 kOe) and \((BH)_{max}=71.64\ kJ/m^{3}\) (Clark, Belson, 1972; Clark, 1973). A. N. Koon, et al. found high coercivity in amorphous alloy \(La_{5}Tb_{5}(Fe_{80}B_{20})_{90}\) in 1981 (Koon, Das, 1981). H. H. Stadelmaier, et al. found that \(Gd_{2}Fe_{20}C\) phase had the similar structure with \(Zn_{2}Cs\) in 1981. Successfully, G. C. Hadjipanayis, et al. confected \(Pr_{16}Fe_{76}B_{8}Si_{2}\) and acquired \(_mH_{c}=1194\ kA/m\) (15kOe) and \((BH)_{max}=103.5\ kJ/m^{3}\) (13MGs·Oe) through fast quenching and heat treatment process (Had-jipanayis, Hazelton, Lawless, 1984). D. J. Sellmyer, et al. found that alloy hard magnetization phase was \(RE_{2}Fe_{14}B\) phase using X - ray analysis, which belonged to square structure compound.
Birth of the third generation rare earth permanent magnetic alloys realized the desire people expected for a long term not only because it created the highest re-cord in magnetic - energy product but also because using neodymium with a higher reserves to substitute samarium with the reserves much fewer, and using iron to substitute cobalt the strategic material. Thus permanent magnet can be used in much wider domains with a better performance and cheap material without any resource limitation. Developing, manufacturing and using the third generation rare earth permanent magnetic alloy was aroused strongly in industrial and aca-demic community, which rapidly changed the situation of research, manufacture and application of rare earth permanent magnetic alloys.
In 1985 the magnetic - energy product of NdFeB alloy reached \(372.92\ kJ/m^{3}\) (47.1 MGs·Oe) already. In 1988 Sumitomo Special Metals Corporation of Japan reported the highest performance of a new NdFeB material that \((BH)_{max}=46.24\ kJ/m^{3}\) (55.78 MGs·Oe), \(B_{r}=1.514\ T\) (15.14kGs), and \(_mH_{c}=694.4\ kA/m\) (8.6 kOe) (Wang, 2001).
In 2002 laboratory of VAC corporate in Germany produced sintered NdFeB permanent magnet with magnetic energy product reaching \(451.3\ kJ/m^{3}\) (56.7 MGs·Oe). Afterwards, Nippon Sumitomo corporate raised the maximum magnetic energy product to \(460.1\ kJ/m^{3}\) (57.8 MGs·Oe) in 2005 and produced a sintered magnet of NdFeB with the maximum magnetic energy product \((BH)_{max}=474\ kJ/m^{3}\) (59.5 MGs·Oe) in 2006, the other magnetic parameters of this sintered magnet: \(B_{r}=1.555\ T\), \(_mH_{c}=653\ kA/m\).
That we called NdFeB magnet as a king of permanent magnetic material is in a certain condition because the Curie temperature of the ternary NdFeB alloy is only \(312^{\circ}C\) so that it is suited to be used in a working environment below \(100^{\circ}C\) and when ambient temperature is over \(100^{\circ}C\) its thermo - stability becomes worse than \(SmCo_{5}\) and Sm - Co of 2:17 type. In other words, at higher temperature the practicability of this kind of magnet is influenced by the following three factors: irreversible loss of magnetic flux, reversible loss of remanence \(B_{r}\) and reversible loss of intrinsic coercivity. The irreversible loss with low coercivity represents considerable severe and even the high magnetic - energy product loses its advantage of the already obtained high magnetic - energy product after tempera-ture circulation (from low temperature to big temperature and again return-ing to room temperature) and causes big change in operating point of magnetic circuit design because of low coercivity. The design of magnetic apparatus re-quires permanent magnet to provide a constant magnetic flux and does not allow the magnetic flux to vary apparently with change of temperature. In the design of magnetic circuit of magnetic apparatus and precise instrument generally adopts a brief interior compensation (using low \(\alpha\) compensating magnet) to ensure a con-stant magnetic flux, while sometimes external compensation is also adopted (add-ing compensation material to out of magnet) (Pan, Chen, Liu, et al, 1994).
It was found in experiment that the Curie temperature of \(Nd_{2}Fe_{14}B\) was mainly indicated by the \(RE = Gd\) as the maximum and then lowered to sides of higher and lower of the atom ordinal in sequence. Using cobalt to substitute part of iron can enhance Curie temperature, such as \(Nd_{2}(Fe_{1 - x}Co_{x})_{14}B\) when \(x = 0.1\) the alloy is of the maximum theoretical magnetic - energy product but afterwards found addition cobalt alone lowered constant of anisotropy of \(Nd_{2}Fe_{14}B\). Addition of cobalt and some other components simultaneously achieved good result, such as NdFeCoGa alloy could raise Curie temperature of NdFeB alloy from \(312^{\circ}C\) to \(450 - 500^{\circ}C\) and \(Nd_{15}Co_{6}Fe_{66 - x}Ga_{2}B_{7}\) at \(x = 2\) the intrinsic co-ercivity of the alloy peaked the value. Its thermal stability improved and be-cause of adding gallium the temperature coefficient of \(B_{r}\) and intrinsic coercivity \(H_{c}\) become smaller.
Furthermore, the coercivity and irreversible loss can be improved by using aluminum, niobium, molybdenum and tungsten to substituting part of iron. The Curie temperature of NdFeB can be raised from \(312^{\circ}C\) to \(450^{\circ}C\) by cooperative using aluminum and cobalt to substitute part of iron. Using \(4\%-10\%\) of cobalt to substitute part of iron could raised the Curie temperature to \(440^{\circ}C\) and if the per-cent of cobalt is raised to \(16\%\) and adding a little aluminum (such as \(2\%\)) can raise Curie temperature to \(480 - 500^{\circ}C\) and decrease the reversible temperature coefficient significantly to \(\alpha_{Br}=0.04\%/^{\circ}C\).
M. Tokunaga, et al researched the result using niobium to substitute part of iron in NdFeB. The result indicated that: addition of niobium in NdFeB alloy made the reversible temperature coefficient of \(B_{r}\) be lowered by \(7\%\) and \(\beta\) the reversible temperature coefficient of \(H_{c}\) be reduced by \(1\%\) (at temperature range of 23 - \(125^{\circ}C\)). The granule size of NdFeBNb alloy decreased along with increasing of niobium content and that the irreversible loss declined obviously along with reducing of average crystal granule size.
In order to improve thermal stability generally a great lot of efforts have been focused on enhancing coercivity. Using dysprosium to substitute part of neodym-ium may be a way to enhance coercivity. Researches indicated that although using dysprosium to substitute part of neodymium can lower \(T_{c}\) yet \(\alpha\) is declined. The main reason is that dysprosium substitution partly compensates degradation of magnetic moment of neodymium. Using a little dysprosium to substitute part of neodymium and controlling temperature system could improve \(H_{c}\) up to above \(1600\ kA/m\) (20×10⁴Oe)(Cai, Rong, 2012; Pan, Ma, Ping, et al, 1991).
To lower temperature coefficient of NdFeB alloy using erbium and dysprosium to partly substitutes neodymium of NdFeB alloy can obtain good result.
Irreversible loss of \((Nd_{0.94}Dy_{0.06})_{16}(Fe_{0.93}Co_{0.05}B_{0.08}Nb_{0.015}Ga_{0.045})_{77}B_{7}\) magnet is less than 5% after exposed at \(260^{\circ}C\). \((Nd_{0.88}Dy_{0.12})_{16}Fe_{77}Co_{0.05}Al_{8.5}B_{7}\) magnet has pref-erable performances: \(B_{r}=1.19\ T\), \(_{i}H_{c}=1630\ kA/m\), \((BH)_{max}=275\ kJ/m^{3}\) (Liu, Pan, Luo, et al, 1991; Pan, Chen, Liu, et al, 1994).
Great deals of corrosion resistant research have been done on the weak point being rust easily of NdFeB magnet under certain conditions.
In 1988, J. Jacobson, et al presented their research result about oxidation reac-tion of NdFeB, NdDyFeB and NdFeAlB alloy at different temperatures in oxida-tion environment. The thesis pointed out: when magnet was exposed in the humid atmosphere at room temperature oxides occurred at edges and corners of the magnet but dispersed over its surface discontinuously. Under dried atmosphere at \(150^{\circ}C\) corrosion phenomenon also took placed and corrosion occurs at the inter-granular boundary. It was determined by X - ray analysis that the oxide in major part composed of ferric oxide and its chemical reaction equations are as follows:
Fe + 1/2O2= FeO
Fe + 2H2O = Fe(OH)2 + H2
In the humid atmosphere and at \(150^{\circ}C\) the chemical reaction equations are:
2Nd+3/2O2 = Nd2O3
2NdCl3 + 3H2O=Nd2O3 + 6HCl
It can be seen that most of oxides turning to \(Nd_{2}O_{3}\). That \(2NdCl_{3}+3H_{2}O = Nd_{2}O_{3}+6HCl\) indicates the specimen being polluted by chloride. At room tem-perature or at \(150^{\circ}C\) but in humid atmosphere oxidation kinetics follows the pa-rabola rate equation that increment in weight increases along with rising of tem-perature. Whereas in drying atmosphere at \(150^{\circ}C\) the weight increment is faster than that in humid atmosphere and a simple parabola rate law is not followed here.
According to the researches of A. S. Kim, et al., adding aluminum and dyspro-sium in NdFeB alloy could also slow down the rate of oxidation at room tempera-ture in the humid atmosphere.
C. N. Christodoulou, et al pointed out based on their researches that powder was oxidized at low temperature when powder was heated (in sintering process).
According to the researches of N. Inaizumi characteristics of magnet became damaged and easily corrodible after mechanical processing. But the damaged magnetic characteristics was restored after heat treatment at \(600 - 1000^{\circ}C\). Further detection discovered the reason for the damage that the oxide layer of about \(0.3 \mu m\) formed to the surface after mechanical processing. Corrosion phe-nomenon did not happen to the magnet when it is exposed in the atmosphere for 100 h (in condition of \(60^{\circ}C\) and \(90\%\) relative humidity).
In order to prevent magnet from corrosion by oxidizing or oxides people adopted two countermeasures: one is to add a little chromium or nickel to im-prove corrosion resistance; the other one is to add protective coating on surface of magnet.
According to report of U. C. Standiego and Osterrecher oxidation rate of \(Nd_{2}Fe_{14}B\) lowered from one half to one third in comparison with \(Nd_{2}Fe_{14}B\).
Anticorrosion coatings means coating of nickel, aluminum, chromate and epoxy resin, etc. and uses different coating according as different purpose. Not all the anticorrosion criterions are the same for different countries. That used in Japan is stricter than that in USA. It should be pointed out that anticorrosion coating is not perfect and the metallic coating would begin to be spolt from surface of mag-net after holding at atmosphere for 2 - 3 years but electroplating or chemical coat-ing is easy to have acidic or alkaline solution remained in pores of magnet. Resin coating is the better way in a severe corrosive environment.
Domestic Advancements in Rare Earth Magnet Technologies
China has abundant rare earth resource and its reserves and output are both in the top of the world, which is advantageous for the development of rare earth perma-nent magnetic alloys. The development of rare earth permanent magnetic alloys in China begins in 1980s. Early in 1970 both Beijing General Research Institute of Iron and Steel and the Beijing General Research Institute of Nonferrous Metals could supply \(SmCo_{5}\) in small amount and successively Baotou Research Institute of Rare Earth, Southwest Applied Physics Research Institute and some other units developed \(PrCo_{5}\) respectively. And the \(H_{A}\) of compound of \(PrCo_{5}\) reached \(11,542 - 14,328\ kA/m\), its magnetic induction intensity was up to \(\mu_{0}M_{s}=1.25\ T\) (\(4\pi M_{s}=12.5\ kGs\)) and its theoretical magnetic - energy product was up to \(310.4\ kJ/m^{3}\). Through its performance was lower than that of \(SmCo_{5}\) but the reserves of Pr is 2 - 4 times of the reserves of Sm in rare earth mines. When developing \(SmCo_{5}\) people already concerned the development of \(PrCo_{5}\) permanent magnetic alloy. The Curie temperature of \(PrCo_{5}\) is close to that of \(SmCo_{5}\). \(SmCo_{5}\) has a higher magnetic anisotropy but \(PrCo_{5}\) has a higher \(M_{s}\) and the resource of Pr is abundant than that of Sm so that taking both advantages made (Sm, \(Pr)Co_{5}\) by using Pr to substitute Sm in powder metallurgic method. Owing to its good magnetic performance and a better economy prospect \((Sm, Pr)Co_{5}\) has been produced in a great deal and used widely. Successively liquid phase sintering process was adopted and its magnetic characters were enhanced in large magnitude. The mag-netic characters of alloy of composition \(Pr_{0.5}Sm_{0.5}Co_{5}\) was up to \((BH)_{max}=159.2\ kJ/m^{3}\), \(B_{r}=0.89\ T\) and \(_iH_{c}=1154\ kA/m\) after solid phase sintering and its maxi-mum magnetic energy product was up to \(199\ kJ/m^{3}\) but its performance at low temperature was far from as good as that of the \(SmCo_{5}\).
It was found from the elements periodic table that Ce is close to Pr and Ce is cheaper than Pr and \(SmCo_{5}\) and its resource is abundant so that \((Ce, MM)Co_{5}\) was developed successively in China. In order to reduce cost further \(Ce(Co,Cu,Fe)_{5}\) permanent magnetic alloy was developed successively using iron and copper to substitute part of expensive cobalt. Through the magnetic performance of the newly developed alloy was as good as \(SmCo_{5}\) and \((Pr, Sm)Co_{5}\) its still reached magnetic performance as follows: \(B_{r}=0.6 - 0.8\ T\), \(H_{c}=860 - 1114\ kA/m\) and \((BH)_{max}=83 - 138\ kJ/m^{3}\).
It was found in practice application that the above mentioned products could not satisfied the requirement for the application of traveling - wave tube, magnetic bearing and precision instrument because their reversible temperature coefficient of magnetic induction is on the high side. The requirement for application situa-tion of above products asked the magnetic induction reversible temperature coef-ficient of permanent magnetic alloy is less than \(-0.02\%/^{\circ}C\). How to lower the temperature coefficient of the above mentioned alloy? It was found through re-searches that the magnetic induction reversible temperature coefficient of \(RECo_{5}\) is mainly determined by the dependency relationship of magnetic moment of molecule constituted it and temperature. In \(RECo_{5}\) the light rare earth metals (La, Ce, Pr, Nd, Sm and Eu) atomic magnetic moment with the atomic magnetic mo-ment of Co is the ferromagnetic coupling and it has negative temperature coef-ficient. The atomic magnetic moment of heavy rare earth metals (Gd, Tb, Dy, Ho, Er, Tm, Yb and Lu) with atomic moment of Co is ferromagnetism cou-pling, which has positive temperature coefficient within a certain temperature range. Both of them have the function of temperature compensation. Therefore, using light rare earth metals partly and heavy rare earth metals partly as RE, in \(RECo_{5}\) alloy can obtain the permanent magnetic alloy with low temperature coef-ficient \(RECo_{5}\). \(Sm_{0.9}Dy_{0.1}Co_{5}\) was made with the maximum magnetic energy product \(72.4\ kJ/m^{3}\) and the magnetic induction reversible temperature coefficient \(-0.0003\%/^{\circ}C\) at \(22 - 47^{\circ}C\) through researches and that by adjusting the ratio of the light and heavy rare earth metals can also obtained an alloy with zero - temperature coefficient.
From the end of 1970s to the beginning of 1980s domestic researchers working on rare earth permanent magnetic alloy systematically developed \(Sm(Co, Cu, Fe, Zr)_{z}\) (\(z = 7 - 8\)) closely following overseas development of Sm - Co 2:17 type permanent magnetic alloy. Beijing University of Science and Technology, Beijing Gen-eral Research Institute for Nonferrous Metal, Institute of Electronics of China Academy of Science, etc. made many systematic researches on the performance of permanent magnetic alloy, especially the mechanism of coercivity. In 1980, D. Li group of Beijing General Research Institute of Iron & Steel successfully devel-oped Sm - Co 2:17 type permanent magnetic alloy with low temperature coefficient with the magnetic performance as follows: for \(Sm_{1.6}E_{0.8}Co_{10.5}Cu_{1.5}Fe_{2}Zr_{0.2}\) alloy average temperature coefficient within temperature range from \(50^{\circ}C\) to \(100^{\circ}C\) \(\alpha=-0.006\%/^{\circ}C\), \(B_{r}=0.99\ T\), \((BH)_{max}=179.8\ kJ/m^{3}\); and for \(Sm_{1.6}E_{0.8}Co_{10.5}Cu_{1.5}\) \(Fe_{2}Zr_{0.2}\) average temperature coefficient \(\alpha = 0.000\%/^{\circ}C\) and within temperature range from \(50^{\circ}C\) to \(100^{\circ}C\) \(\alpha=-0.002\%/^{\circ}C\), \(B_{r}=0.94\ T\), \(H_{c}=413.9\ kA/m\), \((BH)_{max}=143.2\ kJ/m^{3}\). In 1987 J. Wang from Baotou Rare Earth Research Insti-tute systematically studied on \(Sm_{10}Zr_{0.25}(Co, Cu, Fe, Zr)_{17.4}\) permanent magnetic alloy with magnetic performance: \(B_{r}=0.906\ T\), \(H_{c}=1018.8\ kA/m\), \(_iH_{c}=636.8\ kA/m\), \((BH)_{max}=141.8\ kJ/m^{3}\).
After long time researching, the General Research Institute for Nonferrous Metals worked out \(SmCo_{5}\) powder by reduce - diffusion method; Yu Chengzhou, Ying Qimin group together with Gao Qinghai, et al for Shanghai Yuelong Chemical Plant mastered the key techniques for industrialization and set up \(SmCo_{5}\) alloy powder factories. In 1980 to 1983, Tang Renyuan, Li Guokun, et al proclaimed application result of rare earth permanent magnetic material in electric machine, magnetism transmission, magnetism biology and electronic industry; and Sun Tianduo, Sun Daku, et al presented theoretical investigation results on coercivity mechanism of \(SmCo_{5}\) and Sm - Co 2:17 alloy in the international sci-ence conference, which promoted improvement in magnetic performance of rare earth permanent magnetic materials and exploited application scopes for rare earth permanent magnetic materials. In 1983, Pan Shuming subject group prom-ulgated in International Rare Earth Permanent Magnetic Material Academy Con-ference that the observation on variation of \(Sm_{2}Co_{7}\) phase at high temperature for first using JEM - 1000 HVEM in Beijing General Research Institute for Nonfer-rous Metals, and, together with Jin Hannan from Jilin University, presented opin-ions on that coercivity of \(SmCo_{5}\) decreased at the temperature of \(750^{\circ}C\) is because defects in precipitated 2:17 phase which is of a low magnetic anisotropy and so that leads to degradation of the coercivity.
The development of iron base rare earth permanent magnetic alloy is in 1980s, while the broad scale research began in 1983. Some innovations are as follows:
In 1986, Li Wei subject group, Beijing General Research Institute for Iron and Steel, proclaimed that the permanent magnetic alloy was prepared using heavy rare earth metal dysprosium to substitute part of Nd and using iron to substitute part of Co with an average coefficient of magnetic induction being lower than
−0.028%/°C measured at temperature of \(20 - 100^{\circ}C\), and its magnetic performance being: \(B_{r}=0.95\ T\), \(_iH_{c}=1200\ kA/m\), \((BH)_{max}=160\ kJ/m^{3}\). Also, they developed NdFeB alloy with zero temperature coefficient and its magnetic performance be-ing: \(B_{r}=0.79\ T\), \(_iH_{c}=1280\ kA/m\), \((BH)_{max}=114\ kJ/m^{3}\). In 1988, Zhou Shou-zeng subject group of University of Science and Technology Beijing proclaimed \((Nd_{0.5}Dy_{0.03})_{15.5}Fe_{73.5}Co_{2}B_{7.5}\) permanent magnetic alloy with \(\alpha = - 0.000\%/^{\circ}C\) (i.e., the ferrous base rare earth permanent magnetic alloy with zero temperature coef-ficient), within \(20 - 100^{\circ}C\) and the magnetic performance: \(B_{r}=0.88\ T\), \(_iH_{c}=1233.8\ kA/m\), \(_mH_{c}=525.4\ kA/m\), \((BH)_{max}=119.4\ kJ/m^{3}\).
Among high performance permanent magnetic materials the most attractive one is the high coercivity Nd - Fe - B permanent magnetic material because the rema-nence and magnetic energy product of sintered Nd - Fe - B permanent magnet have reached 93% of the theoretical value but its coercivity only reaches 12% of the theoretical value, i.e., 12% of the anisotropic field of compound \(Nd_{2}Fe_{14}B\). Therefore, there is a large space for upgrading of coercivity. Accordingly, the rare earth permanent magnetic material of high coercivity and high performance without using heavy rare earth element will become a new and attractive hot sub-ject for study.
The research of ferrous - base rare earth alloy has been improved in China. In the resent more than 10 years State Intellectual Property Office promulgated in-novation patents, such as “The method of preparation of single - phase intermetallic compound and product”, “Carbide permanent magnet” (Luo, Dong, 1999; Luo, Dong, 1998; Luo, Dong, 1997); “The manufacturing method of permanent mag-netic material”, “The manufacturing process of thorium - manganese 12 type rare earth - iron permanent magnetic material”; “The fabrication of neodymium iron permanent magnet alloy in precipitation - restoration diffusion process”; “A method of producing Sm - Fe - N permanent magnetic alloy powder in reduce - diffusion process”; “High - performance bidirectional rare earth permanent mag-netic material and its preparation method”; “A kind of high stable rare - earth - iron permanent magnet carbide and its manufacturing method”; “A method of fab-ricating hydrogenation - disproportionation - dehydrogeneration - reform rare earth permanent magnetic powder”; “A kind of preparation method of permanent mag-netic powder”, etc.
Pan Wei, et al successfully developed radiation orientation magnetic ring. In recent years in domestic rare earth permanent magnetic products industry rapid coagulating technology, the polarization of hydrogenation milling technology and one - step forming instead of two - steps forming were adopted to increase product density and promote product quality. Chinese rare earth permanent magnetic alloys reaching overseas advanced level.
The production output of rare earth permanent magnetic alloys in China go upon the top stage was not divided from the industrialization policies promoted by academician Wang Zhenxi, et al. In the twenty first century, China should take full advantage of advantaged rare earth resource and promote the rare earth per-manent magnetic alloy product to go up to advanced status in the world.
There is a lot of equipment in developing melting technique for high perform-ance sintered Nd - Fe - B magnet in China.
General Research Institute for Nonferrous Metals is a largest - scale comprehen-sive research and development institution in nonferrous metals, the rare earth metallurgy and material, micro - electronics and photolectron, and rare metal and noble metal materials. It has 10 national levels attached institutions such as Na-tional Engineering Research Center for Rare Earth Material, etc. National Engi-neering Research Center for Rare Earth Material is the core of research and de-velopment of General Research Institute for Nonferrous Metals, which applied 206 patents about the rare earths, include 187 invention patents, 9 overseas pat-ents. It is one of enterprises firstly developed \(SmCo\) permanent magnet in domes-tic in 1970s. Its \(SmCo\) permanent magnet was applied to the 1st artificial satellite of China and made contribution to Chinese “A - bomb, H - bomb and artificial satel-lite”. National Engineering Research Center for Rare Earth Material independ-ently developed the 1st belt throwing off furnace for rare earth \(NdFeB\) alloy which broke through the key technique for quick cooling thick belt of high per-formance \(NdFeB\) (quick solidified casting belt), obtained 4 invention patents and successfully realized mass production, that made important contribution to let Chinese \(NdFeB\) industry enter into high - end application field broke through mo-nopolization of foreign countries and obtain the 2nd grade national invention award.
Since 2003 Ningbo Konit Industry Co. Ltd. became a leader in production of high end product of the rare earth permanent magnetic alloy in application - volume coil motor (VCM) for computer hard disk driver. Their productivity reached thousands tons each year since it produced sintered \(Nd - Fe - B\) magnet and these products have entered into markets of developed countries such as Japan and Europe markets.
Beijing Sanjili Rare Earth Co. Ltd. has mastered excellent casting and solidifi-cation technique and near fast solidification squamous technique (SC), had 4 ad-vanced vacuum induction furnaces with thousands tons output of \(Nd - Fe - B\) annu-ally and became a domestic classic manufacturer.
Ningbo Ninggang Permanent Magnetic Material Co. Ltd. undertakes specially development and production of sintering 1:5 and 2:17 Sm - Co magnetic materials. It has advanced production, machining and inspection equipment and facilities for annual yield of 250 t Sm - Co magnetic materials and the quality of its products reach the advanced level of the world. Ningbo Ninggang Permanent Magnetic Material Co. Ltd. independently developed 1:5 and 2:17 Sm - Co permanent magnetic materials with low temperature coefficient which can be widely used in aerospace, military transducers, etc. It is the largest enterprise of the world in scale of production of Sm - Co permanent magnet.
As one of fast - growing rare earth magnet manufactures, Feller Magtech spe-cialized in \(NdFeB\) permanent motors used in oil pumps, water pumps in coalfield and direct driven direct wind power generators.
Survey of Preparation Technologies for Rare Earth Permanent Magnets
For many years technique of powder metallurgy method, alloy melt rapid solidifying method, diffusion reduction method, etc. to prepare \(NdFeB\) permanent magnetic material achieved quiet great progress. The process flow diagrams of several methods are shown in Fig. 5.1.
Alloy melt rapid solidifying method: USA is famous for manufacturing \(NdFeB\) by this method. GM Corporation used this method to produce \(NdFeB\) permanent magnetic materials. IG Technologies, Ovonics Synthetic Materials Company, Har-rison Dickson, etc. also used this method. For example, the powder of MQ3/4 of GM Corporation exported to Japan was produced using this method. The main steps of this method are mainly as follows: melting neodymium, iron and boron in high - frequency electric furnace, afterwards ejecting to water - cooled copperplate for quenching, thus obtained the alloy strip with a thickness approximate 20μm, and after that grinding the alloy trip to fine powder. According to process flow in Fig. 5.1, processing and further producing goods as requested.
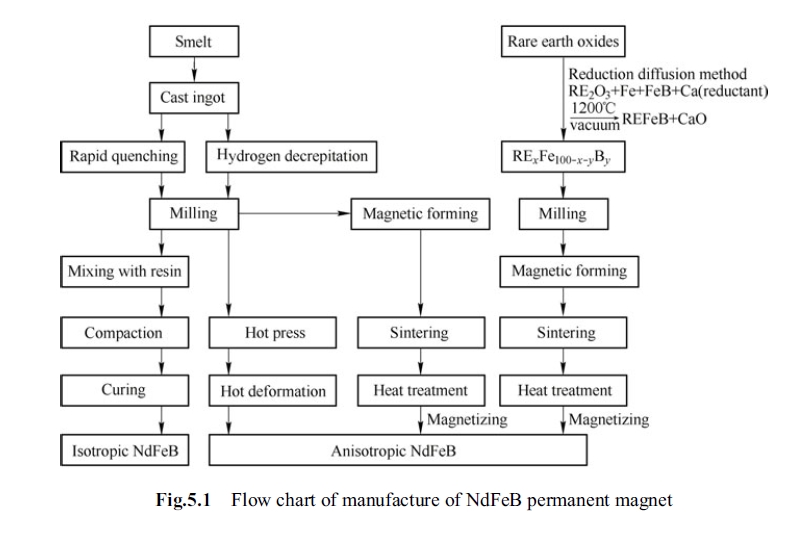
China began to make broad researches on this method since 1983, created its own features with bold innovations, and obtained independent patents of China. The \(NdFeB\) compound factories have been built independently in China, which can provide magnet of any shape for customers.
Powder metallurgy method: currently 80 - 90% of \(NdFeB\) permanent magnetic alloy has been manufactured by this method. Compared to alloy melt rapid solidi-fied method, this method needs less investment and get higher magnetic energy product. Its process flow diagram is shown in Fig. 5.1. That is, raw material \(\to\) alloy smelting(medium - frequency induction furnace) \(\to\) milling \(\to\) forming in magnetic field \(\to\) sintering \(\to\) aging (heat treatment) \(\to\) magnetization \(\to\) anisot-ropic \(NdFeB\) permanent magnetic alloy. Because neodymium is easily to be oxi-dized, the key technology is protecting against oxidation. Vacuum pumping and inert gas protecting is necessary for alloy smelting and sintering. China has got-ten several intellectual properties and patent rights by bold innovation in technol-ogy research.
The sintering process plays a decisive role in the manufacturing process of high - performing rare earth iron - boron base materials. The purpose of sintering is to make sure that the migration of atoms at high temperatures occurs between powder particles bonded to the alloy performance quantitative and qualitative transformation, bond strength between the powder alloys to meet the require-ments. Sintering process of diffusion, flow, re - crystallization, creep, reply materi-alized is almost the same time (Pan, 2011).
Diffusion reduction method: in Symposium of International Rare Earth Perma-nent magnetic Materials and Application Convention opened in May 1985, C. Herget of Goldschmidt Co. in the former West Germanic reported the technology and theory of producing \(NdFeB\) permanent magnetic alloy with rare earth oxide in laboratory.

Goldschmidt Co. had produced \(NdFeB\) permanent magnet with the maximum magnetic energy product of \(167\ kJ/m^{3}\) but a low intrinsic coercivity. By using dyspro-sium to substitute part of neodymium and using aluminum to substitute part of iron the intrinsic coercivity was increased sharply. The intrinsic coercivity of \(NdDyFeB\) alloy was 708 kA/m and the maximum magnetic energy product was \(237.2\ kJ/m^{3}\). The advantage of this method is low cost. Neodymia reacts with reducer and be reverted to neodymium in this process. When inert atmosphere is heated up to \(1200^{\circ}C\) neodymium is inter - diffused with iron and boron to become \(NdFeB\) permanent magnetic alloy. The coercivity of magnet in this method is lower than those made by powder metallurgy method and alloy melt rapid so-lidified method. This method is still in development.
Hot pressing and hot rolling method:Main procedures of this method are: the Pr(Nd)FeCuB alloy ingot → enclose with metal → heating → hot machining, and then the permanent magnetic alloy with magnetic anisotropy is obtained through hot pressing and hot rolling. Some results have been achieved in China using this method, and permanent magnetic alloys with a maximum magnetic energy product \((BH)_{max}\geq240\text{ kJ}/\text{m}^3\) have been produced.
NdFeB permanent magnetic alloys could be produced by spark plasma sinter-ing technique. Spark Plasma Sintering (briefly called as SPS) is use plasma to be conductive spear inside sample to form electric current, moving directionally under the effect of electric field (plasma swathed on particle surface), merging together at particles connecting node (local current density increase), and forming high sintering points due to high temperature and thus caused substance transfers by evaporation, diffusion, etc. Sintering points increase gradually and distribute equably so that turns into comprehensive sintering status. Its remanence \(B_{r}\) and the maximum magnetic energy product \((BH)_{max}\) appear at \(780^{\circ}C\), intrinsic coercivity \(H_{c}\) turn up at about \(800^{\circ}C\), heat treatment temperature is \(950 - 1000^{\circ}C\). Its magnetic properties can reach: \(B_{r}=1.15\ T\), \(_iH_{c}=1200\ kA/m\), \((BH)_{max}=262\ kJ/m^{3}\). Compared to traditional powder metallurgy technology, the advantages of SPS are, (1) low sintering temperature, (2) ordinary materials sintering densifica-tion only need 3 - 5min, so has high sintering speed, (3) simple operation and short process flow, and (4) effectively inhibiting grain growth of NdFeB permanent magnetic alloy during sintering process (Xiao, Yue, Wang, et al, 2002).
As the world's largest rare earth magnets supplier and market place, Chinese industry has a big demand for milling equipment, calibration service of magnetic properties and machinery processing equipment. Taiyuan Shengkaiyuan Perma-nent Magnet Equipment Corporation developed special equipment for crushing of NdFeB permanent magnet materials—the rotary hydrogenated pulverizing fur-nace. That is an advanced pulverization technique and can effectively control shape and size of powder. Its main technical index reaches international ad-vanced level and can replace imported equipment. At present more than 120 equipments are used in China.
Beijing Xindake Electric Technology Co. Ltd. undertakes production of milling equipment, including coarse crusher, middle crusher and jet mill with nitrogen gas. Among them the QLMR-T series, whole sealing close circular loop jet mill, occupies 70% of domestic jet mill market. It has advantages of good sealing, cen-tralized distribution of powder size and less impurities.
China National Institute of Metrology (NIM) has developed a serials of com-mercial hysteresisgraphs, named NM2000, for determination of magnetic proper-ties of hard magnet, including hysteresisgraph for measuring hard ferrite and rare earth magnets, hysteresisgraph at high temperature(up to \(500^{\circ}C\)), and mag-netic field scanner for multi - pole magnets, etc. Nowadays, due to the traceable
accuracy, reliability, and price only a fraction of that of the developed countries, NIM2000 serials equipped nearly 90% NdFeB manufacturers in the world.
EDTA volume method was used to determine total rare earth contents in Dy-Fe alloy. Iron interference was eliminated by the addition of fluoride ions. Recovery in 99%-101% and RSD less than 1% were obtained. This method is accurate (Sun, Zhang, Zhang, et al, 2010).
Zhaoqing Dingchen Permanent Magnet Equipment Co. Ltd. is the specialty company producing machinery equipment to process permanent magnets. It has a special equipment development tram and obtained several patents. Its process technology is in leading position in China and reaches to advanced level of the world. It has various processing machines, including: digital controlled tile (C shape) grinder, vertical auto-feed dual work position grinder, auto-chamfer grinder, etc. Thus, its equipment is widely used in domestic market for manufacturing the rare earth permanent magnets and imported to part of overseas market, including Germany, Malaysia, India, etc.
Manufacture of bonded permanent magnetic alloys in recent years the applica-tion fields of bonded permanent magnetic alloys have been enlarged continually in China. Among them the output growth rate of bonding permanent magnetic alloys with highly accurate size and complicated shape (bonded NdFeB permanent mag-netic alloy and bonded SmCo permanent magnetic alloy) grows fastest.
The commercialization of bond NdFeB permanent magnetic alloys started at 1980s. GM Corporation exploited raw material to make bonded NdFeB perma-nent magnetic alloy, and produced rapid quenching NdFeB magnetic powder in 1987. Bonded NdFeB permanent magnetic alloys have formed series products in past more than ten years. The price of raw material for bonded NdFeB permanent magnetic alloys is much cheaper than that of bonded SmCo permanent magnetic alloys. And magnetic properties of bonded NdFeB permanent magnetic alloys are higher, manufacturability is better, and material utilization rate is higher. So bonded NdFeB permanent magnetic alloys take most part of bonded rare earth permanent magnetic alloys. The yield of bonded rare earth permanent magnetic alloys is 3,538 ton in 2004 all over the world, where yield in Japan is 565 ton, in China is 1,350 ton.
The manufacturing method of bonded permanent magnetic alloys uses powder bonding method. Bonded NdFeB permanent magnetic alloys could be manufac-tured by compression forming, injection forming, extrusion forming, and calen-dering forming. The operation procedures of compression forming method are: mixing \(\to\) forming \(\to\) solidification \(\to\) machining \(\to\) coating \(\to\) inspection. Although this method has many operation procedures, the production efficiency and material utilization are higher. The maximum magnetic energy product of bonded NdFeB permanent magnetic alloy produced by Aiwa Steel Group in Japan was \(119 - 159\ kJ/m^{3}\). The maximum magnetic energy product and density of differ-ent bonded permanent magnetic alloys are shown in Table 5.3. The comparison of performances between anisotropic and isotropic bonded NdFeB permanent magnetic alloy is given in Table 5.4.
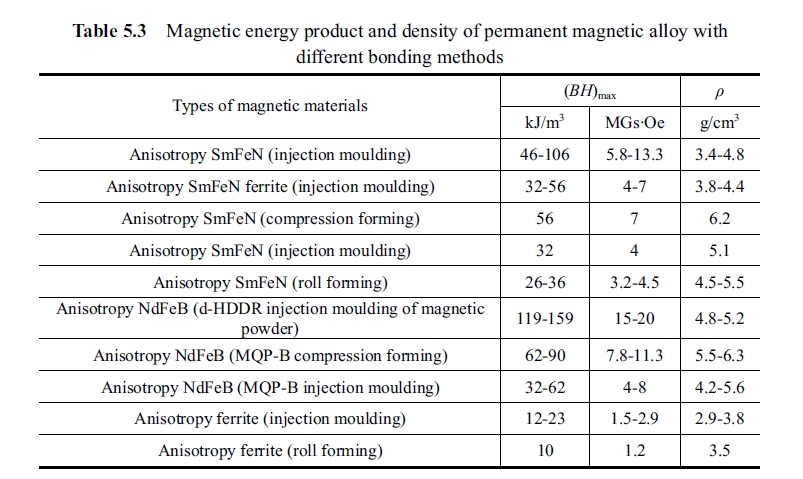
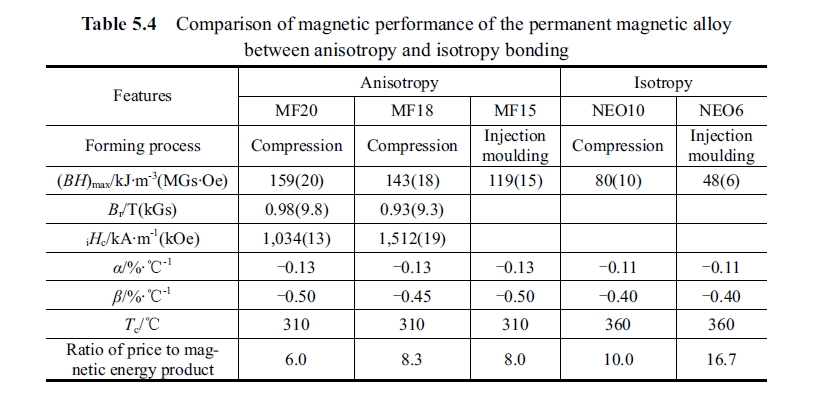
As shown in Table 5.4 anisotropic bonded NdFeB permanent magnetic alloy has higher performance. Because of the development of bonded rare earth perma-nent magnetic alloy technology people could choose different bonded permanent magnetic alloys according to various demands.
In comparison with bonded NdFeB permanent magnetic alloy, sintering NdFeB permanent magnetic alloy has been developing in a high speed in recent years in China. The annual output of sintering NdFeB permanent magnetic alloy reached
83,000 ton in China in 2010. In the last few years the performance of sintering NdFeB permanent magnetic alloy has been improved remarkably as merchandise. Table 5.5 gives the maximum grades of sintering NdFeB permanent magnetic alloy produced by Sumitomo Corporation in Japan. The performances of sintering NdFeB permanent magnetic alloy produced by some Chinese companies reached international advanced standards with maximum magnetic energy product \((BH)_{max}=376 - 408\ kJ/m^{3}\) (47 - 51 MGs·Oe)(Luo, 2003).
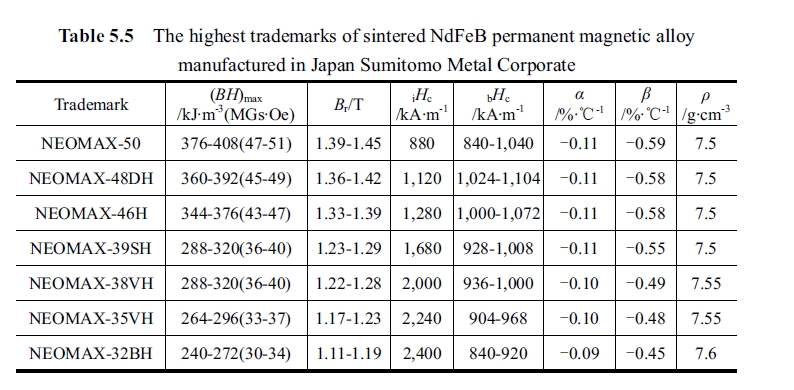
For many years the outstanding evolutions of NdFeB permanent magnetic technology express in undermentioned three aspects: (1) aiming at low Curie temperature and big temperature coefficient of NdFeB alloy, the means to im-prove thermal stability of NdFeB magnet have been considered; (2) in order to overcome easy-rusting of iron base alloy in certain condition anticorrosion re-search have been carried out; (3) respond to requirement of users researches for different materials technique have been developed.
The newly developed heat-flow-transmutation procedures based on heat-pressing technique is an effective way to produce high performance nanocrystal Nd - Fe - B magnet. Process of heat-flow-transmutation is used to precipitate homo-geneous composite magnetic for soft and hard phases of nanometer level from non-crystalline matrix and keeps coherence in interface. That meeting requirement for control microstructure of nanocrystal composite magnet as well as realizing mag-netic anisotropy synchronously provides a way for heat-flow transmutation to produce high performance anisotropic nanometer composite magnet.
Applications and Future Expectations for Rare Earth Permanent Magnet Alloys
The aim of developing new material is for application, but it takes a long time for a new material from exploit to use. And that NdFeB, the king of current permanent magnetic materials, occupied permanent magnet market in a speed without precedent since it was born. Up till now through many years commercializing development it has proved that NdFeB is a very important permanent magnetic material with wide of application fields and large potential. The committee of the european communities has analysed the market of NdFeB and figured out in this report that NdFeB alloy should not only be the substitutor of all permanent mag-netic materials for matching existing devices, but also has been widely used in the new market of superseding electromagnetic and non-electromagnetic designed devices. Table 5.6 is the quantity demanded of NdFeB alloy based on market forecast. The actual quantity demanded is far more than the amount listed in the table. Table 5.7 show the application, distributing field and its variation of NdFeB in China, respectively (Pan, Li, 2000; Pan, Ping, Liu, et al, 2003; Luo, 2003; Pan, 2001).
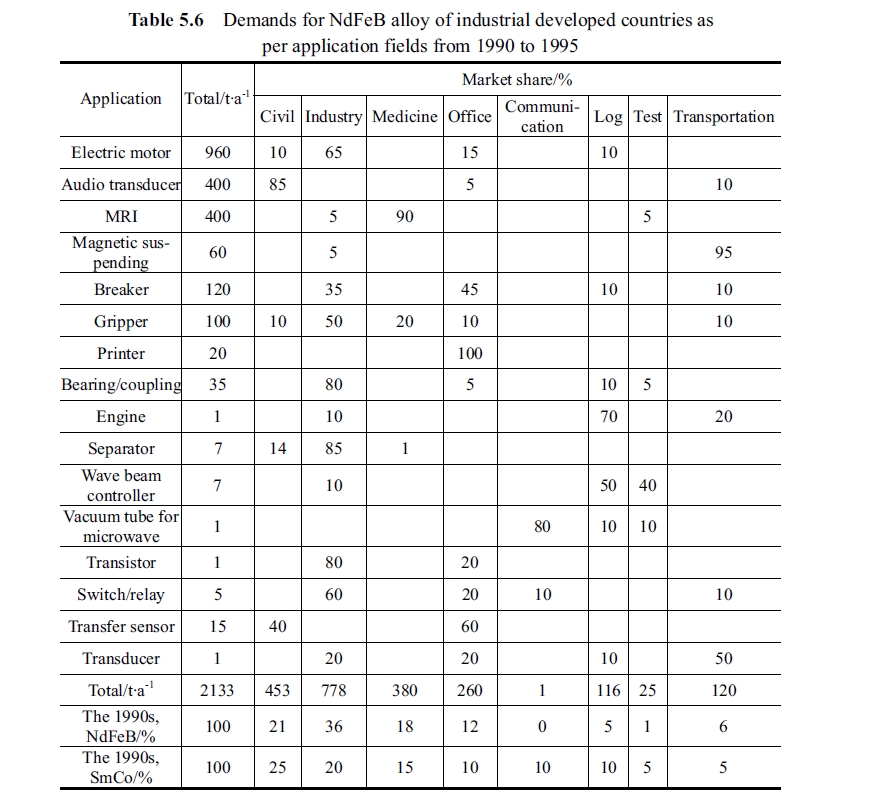
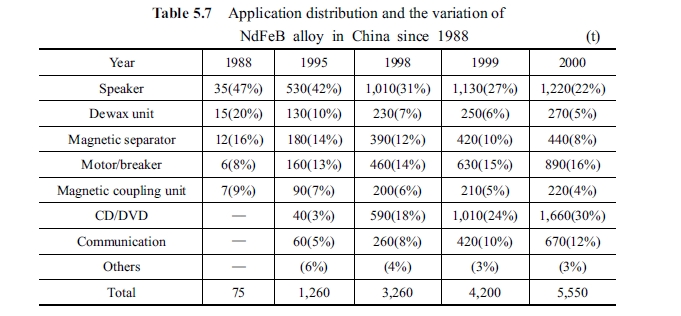
The motors made up with neodymium, iron and boron have the advantages of high efficiency, big unit power, light, small volume, etc. NdFeB permanent mag-netic motors and SmCo permanent magnetic motors do not need electric excita-tion, then they do not have excitation coil and iron core. Thus the volume and quality of these motors can be reduced by more than 30%. And that NdFeB per-manent magnet motors do not have excitation loss so that its efficiency is higher than common motors. Magnet itself does not lose so that in condition of same tem-perature rising NdFeB permanent magnetic motor has large allowable output power, so it has high efficiency. The 5.5 kW synchronomotor used in colliery refit-ted by Northwest Technical University using rare earth permanent magnetic synchronous motor. Afterwards, its efficiency was improved from 74% to 83%, \(cos\varphi\) was enhanced from 0.78 to 0.86. Extension of this motor will save a great deal of energy sources. General Motor Company successfully developed starting motor of automobile with NdFeB permanent magnetic alloy and launched into small batch production in 1986. Benz Corporation also carried out trial-manufacturing successfully. Using permanent magnetic alloys to produce motors began in 1984 in China. In 1985, Northwest Technical University and cooperated with other organization successfully manufactured 1.5 kW startup motor for automobile with NdFeB permanent magnetic alloy. In 10 kW cyathiform armature motor AlNiCo permanent magnetic alloy was replaced by NdFeB permanent magnetic alloy. The weight of permanent magnet alloy reduced from 141g to 45g and the cost of permanent magnet cut down from 28 Yuan to 13 Yuan but the power was enlarged from 4W to 10W. The manufacturing and research in NdFeB permanent magnetic motor developed rapidly in recent years. Since 1987 NdFeB permanent magnetic alloy had been successfully applied in synchronous motor, servo-motor, DC motor and other two types of motors. With the enhancement of thermal stability of NdFeB permanent magnetic alloy, it will develop much faster in the future.
NdFeB permanent magnetic alloy have been successfully applied in MRI. Sub-sidiary companies of Siemens in Germany and China have already sold instru-ments made with NdFeB permanent magnetic alloy.
The application and exploitation of NdFeB material have been developing con-tinuously. Examples of its application based on function show as follows:
Transforming mechanical energy to electrical energy: such as generator, pickup device, geophone, microphone, transmit speed instrument.
Transforming electrical energy to mechanical energy: small motors such as DC motor, trumpets in audio devices, moving - coil instrument, and electromag-netic pump.
Mechanical energy transformation: using attraction of magnet, magnetic cupule, magnetic force transport, magnetic separator, magnetic transmission, magnetic adsorption fixture, piston pump, etc. Using the attractive force and repulsive force between magnets: in magnetic axletree, magnetic puddler, magnetic suspension, magnetic clutch, etc. And using the induced eddy current function between per-manent magnet and conductor: wattmeter, velocimeter, and electric meter, eddy current driving device, etc.
The utilization of magnetic sources: NMR devices, magnetic field generator, dry reed relay, etc.
Deflexion of charged particle in magnetic field: magnetron, traveling - wave tube, electron gun controller, prionotron, electromagnetic flow meter, etc.
Magnetic biology: water magnetizer, magnetotherapy device.
The application in automobile: one modern sedan car has more than 80 parts using permanent magnet. The parts using neodymium - iron - boron permanent magnetic alloy are shown as follows: starter motor, cooling fan, portfire, front light, water meter, driving control device, glass rain scrape, flush pump, motor used in cover sunshine equipment, tape transmitting motor, horn, imitating fuzzy motor, motor used to lock door, retropack, motor used to open and close windows, motor used in fuel pump, motor used to control seats, antenna motor, air condi-tioner, crankshaft transport angle sensor, throttle sensors, velocimeter, etc.
NdFeB permanent magnetic alloy firsty used in automobile was starter motor, in the condition of coequal output torque, weight of motor which is made with NdFeB can be lightened 50%, volume can be reduced 30%-40%, and efficiency can be improved obviously. There are 60 - 80 motors in an automobile. If all mo-tors use NdFeB permanent magnetic motor, more than 5,000 ton bonded and sin-tering NdFeB permanent magnetic alloy would be needed (in demand for 50 mil-lion cars).
Application of NdFeB permanent magnetic alloy in voice coil motor ade-quately incamates on its performance. Voice coil motor is an actua-tor of read - write head of disk drive. Because the trend of miniaturization and even
Micromation of computer voice coil motor requires it to be smaller, lighter, and have the following magnetic capabilities: \(B_r = 1.38\text{T}\), \(_{i}H_c\geq1200\text{kA}/\text{m}\), \((BH)_{max}=368\text{kJ}/\text{m}^3\). The amount used, taking the weight of NdFeB permanent magnetic alloy in one voice coil motor as 15g, requires thousands of tons of NdFeB permanent magnetic alloy to meet the demand.
The biggest amount of NdFeB permanent magnetic alloy is used to manufac-ture permanent magnet motor all over the world. People most need high effi-ciency motors. Thus motor manufacturers make great efforts to enhance efficiency of motors. Motors with different structures have different efficiency (power coef-ficient). The highest efficiency of DC motor is 85%-89%, induction motor is 94%-95%, and permanent magnet motor is 95%-97%. Therefore, permanent magnet motor will take the place of induction motor gradually. Bond NdFeB permanent magnetic alloy takes up the absolute advantage in multipolar spindle motor of hard disk and floppy drive. The required amount is more than a hundred million (Luo, 2002). Recently, according to the requirement of customer, we re-placed NdFeB permanent magnets with Ferrite, AlNiCo and SmCo permanent magnets by using combined geometric circuit design methods to ensure the qualified magnetic properties of the components (Pan, 2011).
Great progress has been achieved in field of applying and developing NdFeB in China. The products with profit over 1 million have: antiwax apparatus, fuel economizer, MRI, magnetic separator, wattmeter, sensor, shock absorber, water magnetizer, magneto therapy apparatus, etc. Apparatuses such as magnetic field generator, magnetron, magnetic stirring, magnetic bearing, magnetic chuck, loud-speaker, etc. have been successfully exploited.
Electric bicycle which is widely used for transportation in middle - small cities get rapid development in recent years. Taking 2002 for example, one million elec-tric bicycles had been produced and nearly 600 ton NdFeB permanent magnetic alloy had been used. Forecasting that in 2007 electric bicycle and power assistant vehicle will need 5,700 ton and 2,385 ton NdFeB permanent magnetic alloy, re-spectively, electric vehicle will need 2,000 ton; total weight will need more than 10,000 ton in one year.
The third generation rare earth permanent magnetic alloy has come out from twenty years, its magnetic energy product has promoted from \(286.6\ kJ/m^{3}\) (36 MGs - Oe) at first to present \(413.92\ kJ/m^{3}\) (52 MGs - Oe) for commercial use. Its yield has increased from a few hundred kilogram to tens of thousands ton (Seen in Table 5.8). The yield of NdFeB sintering permanent magnetic alloy all over the world reached 34,510 ton in 2004. The total yield of bond and sintering perma-nent magnetic alloy is 28,860 ton in China, accounted for 75.26% of world ship-ment (Luo, 2003b). And the prospect of permanent magnetic industry is very good.
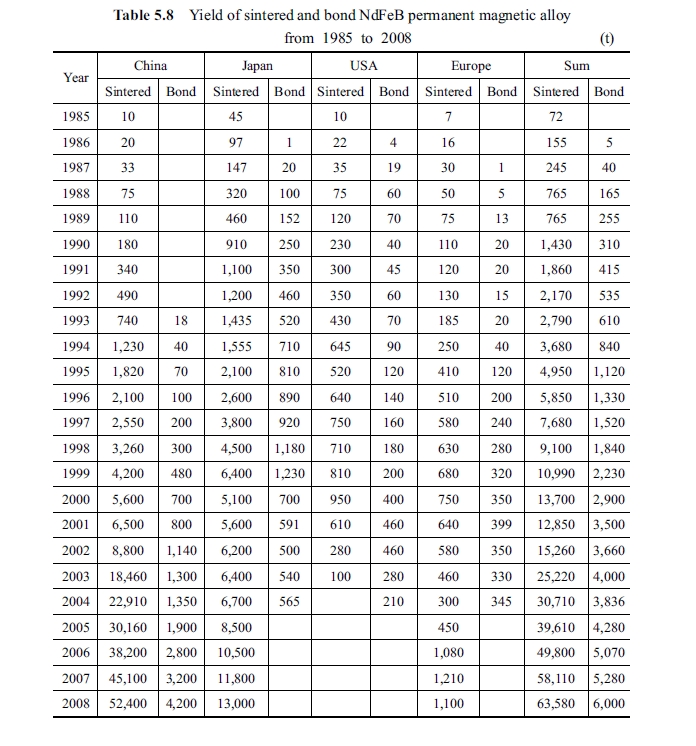