4.10 In Situ and Dynamic Observation on High Temperature Phase Transformation and Magnetism of \(Nd_{16}Fe_{77}B_{7}\) Permanent Magnetic Alloy
NdFeB permanent magnet has been studied widely (Sagawa, Fujimura, Togawa, et al, 1984) since it was found in 1983. Using cobalt to substitute part of iron can raise Curie temperature of the tetragonal phase of \(Nd_{2}Fe_{14}B\) considerably, and can improve thermal stability of the alloy (Sagawa, Fujimura, Yamamoto, et al, 1984; Matsuura, Hirosawa, Yamamoto, et al, 1985; Arai, Shibata, 1985; Hirotsu, Tokuhara, Yamamoto, et al, 1987; Fuerst, Herbst, 1988). Researches indicated that NdFeCoB magnet mainly consisted of quadrilateral phase \(Nd_{2}(Fe, Co)_{14}B\) and phase of crystal interface. The phase of crystal interface contains Nd - rich phase
and Laves phase of \(Nd(Fe, Co)_{20}\) (Arai, Shibata, 1985), and there was report that phase of \(Nd_{20}(Fe, Co)_{80}\) was found as well (Koestler, Ramesh, Echer, et al, 1989). But B - rich phase seems hard to be found. Nevertheless, the remanence is reduced by adding cobalt atom to the tetragonal phase, and that appearance of magnetic soft phase results in dramatically degradation of the coercivity.
Microstructure has important influence on magnetic features, especially on the coercivity, of sintered NdFeB magnet. This section introduces a dynamic observation on microstructure change of \(Nd_{16}Fe_{69}Co_{3}B_{7}\), with optimal magnetic features, at temperature from \(25^{\circ}C\) to \(700^{\circ}C\). Though the microstructure of NdFeCoB magnet has been studied detailedly (Koestler, Ramesh, Echer, et al, 1989), there is no report about dynamic observation. A comparison is made between the dynamic observation result of NdFeB and that of NdFeCoB.
Sample Preparation and Experimental Methods for Nd₁₆Fe₇₇B₇ Alloy Analysis
Neodymium, iron, cobalt and ferroboron with purity above 99.5% were melted in vacuum crucible (\(1.5\times10^{-2}\ Pa\)) to get ingot of the alloy, the ingot was milled to be powder of 3 - 5 μm which was oriented in magnetic field of 1.5 T and was pressed out in axial direction at 2T cm, and then the pressed powder was sintered at \(1100^{\circ}C\) for 1 h and edged at \(550^{\circ}C\). Magnetic measurement indicated that this magnetic possesses outstanding features: its remanence, coercivity and magnetic energy integral were 1.28 T, 589.0 kA/m and 302.4 kJ/m³.
The sintered alloy was sliced to lamella of 0.25 mm in direction perpendicular to \(c\) axis of the magnet for observation under HVM, the lamella was polished and mechanically thinned by ionic beam to prepare film sample which is perpendicular to the axis of magnetic field. The observation was conducted under JEM - 1000 HVM which has temperature controlling unit. Operation voltage was 1000 V.
In-Situ Observation of Nanostructure and High-Temperature Phase Transformation in NdFeB Alloy
The study investigated the behaviors of additional cobalt and the Nd - rich phase at different temperatures, which benefits the understanding of the temperature dependence of the magnetic properties.
High temperature phase transformation of \(Nd_{2}(Fe, Co)_{14}B\)
\(Nd_{2}(Fe, Co)_{14}B\) crystal granule presents as integral crystals at room temperature, which almost exists no defect. Crystal lattice constants of its quadrilateral phase can be calculated by X - ray diffraction pattern as \(a_{0}=0.882\ nm\), \(c = 1.218\ nm\). But in some crystals a few big and many tiny globose impurities distributed in the matrix could be observed clearly, seeing Fig. 4.50(a). These impurities phases were de-
marcated as bcc structure with lattice constant \(a_{0}=1.086\ nm\) by electronic diffraction pattern (Fig. 4.50(b)). They were analyzed as \(Nd_{2}O_{3}\). Fidler, et al also found these impurities in the NdFeB magnet with addition of aluminum and determined them as non - magnetic hexagonal phase of \(Nd_{2}O_{3}\) (Fidler, Knoch, Kronmüllera, et al, 1989). By analyzing their microstructure authors found that there was a non - crystalline phase (Fig. 4.50(c)) between impurities and the matrix. In NdFeCoB magnet the most important ferromagnetic phase is the tetragonal phase \(Nd_{2}(Fe, Co)_{14}B\) which takes on magnetically hard characteristic of magnet, and appearance of the non - ferromagnetic phase and the non - crystalline phase will reduce the remanence of the magnet. In addition, nucleation in non - crystalline phase though reverse domain will make coercivity of the magnet degraded sharply.
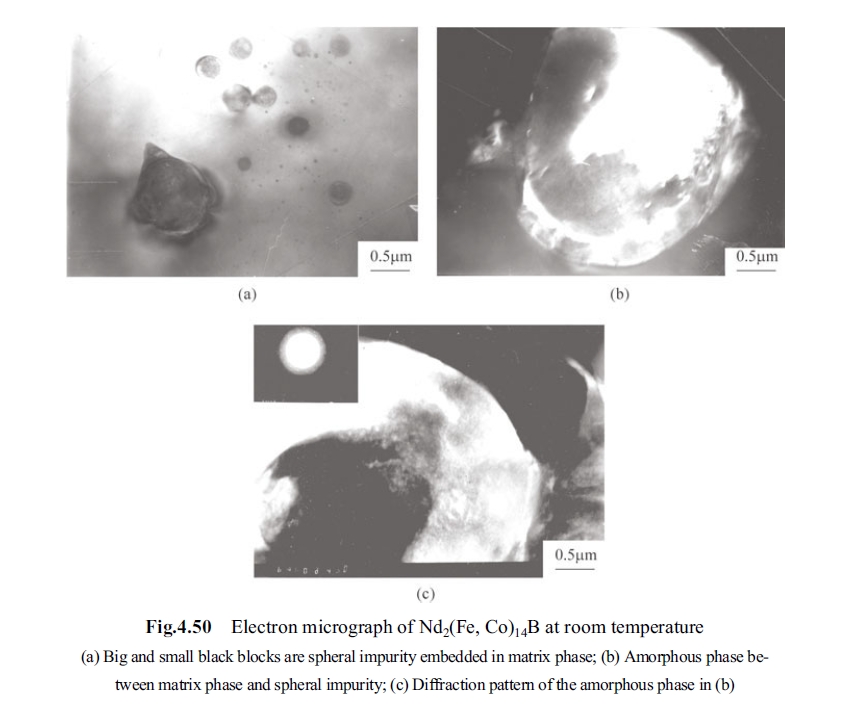
In heating process when temperature was below \(400^{\circ}C\) the microstructure of the quadrilateral phase had no remarkable change; when temperature was higher than \(400^{\circ}C\) some tiny precipitates started to appear in the quadrilateral phase; and when reached \(500^{\circ}C\) the precipitates grew like needle which presented to be per-
pendicular with each other and appeared evenly and largely on the matrix, as shown in Fig. 4.51; when temperature was further raised to \(700^{\circ}C\) the quadrilateral symmetrical the matrix was demolished completely and its microstructure is shown as Fig. 4.52.


Author had conducted the in situ and dynamic observation on \(Nd_{16}Fe_{77}B_{7}\) magnet by HVM and found that the precipitates started to appear in \(Nd_{2}Fe_{14}B\) crystal granule at \(280^{\circ}C\) (Pan, Liu, Luo, 1990). Grössinger, et al had measured that anisotropy field \(H_{A}\) and coercivity field \(H_{c}\) changed with variation of temperature (Grössinger, Krewenka, Kirchmayr, et al, 1987). The \(H_{A}\) and \(H_{c}\) were degraded rapidly along with rising of temperature, degradation of \(H_{A}\) was fastest at around \(280^{\circ}C\) which is the temperature corresponding to the appearance of the main precipitates. It can be seen that the precipitates of the tetragonal phase is the main reason for degradation of \(H_{A}\) and \(H_{c}\) and completed demolition of quadrilateral symmetry will lead to degradation of \(H_{A}\) and \(H_{c}\) to zero.
The precipitates started to appear at \(400^{\circ}C\) in this work and this temperature was about \(100^{\circ}C\) higher than the precipitation temperature of samples without cobalt. It can be seen that addition of cobalt can improve thermal stability of the tetragonal phase. Deeply study on composition analysis and structure ascertainment of precipitated phase would be favorable for developing application of the magnet and understanding of the relation between magnetic features and change of temperature.
The in situ and dynamic observation of crystal interface
The crystal interface phases include Nd - rich phase and a phase of undefined structure. These phases can be observed in crystal interface lamella and interfaces among three or four granules of crystals, seeing in Fig. 4.53(a) and Fig. 4.53(b). The Nd - rich phase at the interface of three granules has fcc structure with lattice constant \(a = 0.55\). The other phase has not been defined because of the complicated electronic diffraction pattern, as shown as Fig. 4.53(b). Many studies indicated that magnetically soft phase of \(Nd(Fe, Co)_{x}\) existed in NdFeCoB magnet, but that whether the phase of undefined structure is belong to the magnetically soft phase still needs further study to determine it (Mizoguchi, Sakai, Niu, et al, 1986).
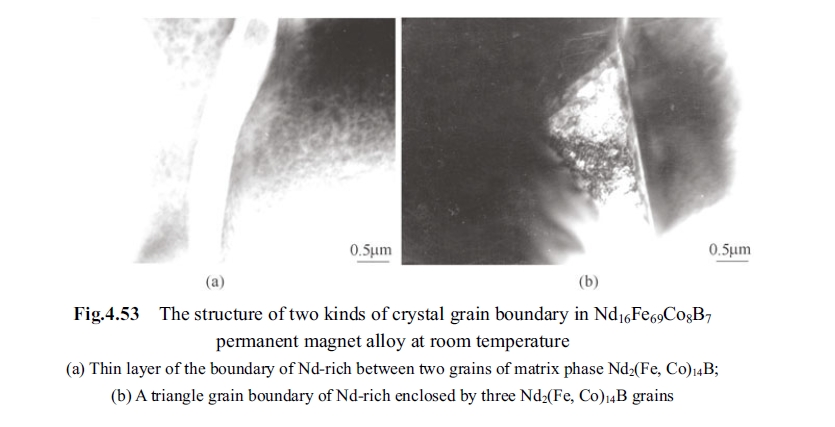
The phase in crystal interface has significant influence on coercivity. The Nd - rich lamella of non - magnetism acts as pinning function to move of the domain wall and so that will enhance the coercivity. But the magnetically soft phase at triangle crystal boundary would act as nucleation center of the reverse domain to produce demagnetization field so that to degrade coercivity sharply. According to the importance of the phase of crystal interface to the coercivity it is necessary to observe them at a high temperature.
The Nd - rich phase and crystal interface lamella phase had no remarkable varia-tion during the whole process of rising temperature. Microstructure of the phase of crystal interface with undefined structure had not remarkable change when temperature raised from \(25^{\circ}C\) to \(600^{\circ}C\). And when temperature was raised to \(700^{\circ}C\) the diffraction pattern of its microstructure is found to have a change. A in Fig. 4.54 is the microstructure of phase of undefined structure at \(700^{\circ}C\), B is the diffraction pattern which appears as multi - crystalline ring. When sample was held for 20 min at \(700^{\circ}C\) the diffraction pattern consisted of a non - crystalline diffusing ring and some diffraction spots, as shown in C. This change process still waits to be studied referring to the result obtained at present. It can be seen from the other group of relationship curve between aging temperature and coercivity of \(Nd_{16}Fe_{69}Co_{3}B_{7}\) alloy (in section 4.10.7) that coercivity of the alloy after sintering was not high, was measured as 379 kA/m; if try to draw a relationship curve between aging temperature and coercivity it can be seen that the intrinsic coercivity of the alloy was the highest as 589 kA/m at \(550^{\circ}C\); the intrinsic coercivity degraded at \(700^{\circ}C\) and enhanced slightly at \(900^{\circ}C\). Ternary NdFeB alloy appeared coercivity cusp at \(600^{\circ}C\), which is considered to be the result of the dissolution of the unstable magnetically soft phase of NdFeB alloy by heat treatment at \(600^{\circ}C\), and this process can improve coercivity of the alloy (Schneider, Hening, Missell, et al, 1990; Pan, Ma, Ping, et al, 1991; Pan, Jin, 1990; Pan, Li, Li, et al, 1989; Pan, Zhao, Ma, 1988). In comparison, experiment by author found the aforementioned process appeared at \(550^{\circ}C\), that is to say, the magnetically soft phase of the alloy with cobalt dissolved at \(550^{\circ}C\). Moreover, the coercivity degraded at \(700^{\circ}c\) considerably, that had no been studied and discussed in reports before. Observation with electronic microscope and the diffraction pattern of the matrix was
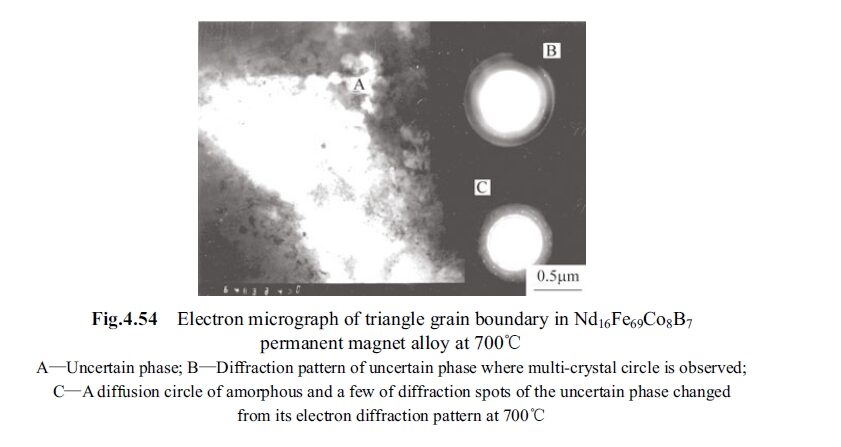
multi - crystal ring at \(700^{\circ}C\), but after holding at \(700^{\circ}C\) for 20 min the diffraction pat-tern changed from the multi - crystal ring to a non - crystalline diffusion ring and some diffraction spots. Accordingly, the coercivity degraded correspondingly at \(700^{\circ}C\).
Role of Cobalt in Enhancing the Performance of NdFeCoB Magnetic Alloys
It was known from measurement result of Curie temperature that using some co-balt to replace part of iron can raise Curie temperature of the alloy. The reason to raise Curie temperature is that the exchange function of Co - Co and Co - Fe is stronger than that of Fe - Fe, and Co occupies \(j_{2}\) and \(k_{2}\) crystal sites preferentially, which can improve negative exchange between \(j_{2}-k_{2}\) sites so that to enhance positive exchange function and weaken negative exchange function.
The \(Nd_{16}Fe_{77}B_{7}\) permanent magnetic alloy mainly consists of the matrix phase of \(Nd_{2}Fe_{14}B\), Nd - rich phase and B - rich phase (\(Nd_{1.11}Fe_{4}B_{4}\)), among of them the phase making the biggest contribution is \(Nd_{2}Fe_{14}B\), accounting for 80% - 85% of the total volume. And \(Nd_{16}Fe_{77}B_{7}\) has the biggest magnetic anisotropy by action of crystalline field because \(Nd^{3 +}\) has track magnetic torque; and the crystalline split of 4f energy grade of the rare earth element is the source of intrinsic magnetic anisotropy of \(Nd_{2}Fe_{14}B\) (Arai, Shibata, 1985). When using some cobalt to replace part of iron the matrix of the alloy becomes \(Nd_{2}(Fe, Co)_{14}B\). To study cobalt the effect of atom of cobalt entering into crystal lattice on Curie temperature \(T_{c}\) a mi-cro - area electron composition analysis was made of the alloy of \(Nd_{16}Fe_{69}Co_{3}B_{7}\). Analysis result indicated that interior of crystal of alloy \(Nd_{2}(Fe, Co)_{14}B\), Fe = 77.3%(at.), Nd = 15.2%(at.), Co = 7.5%(at.); in comparison with micro - area electronic probe analysis a conclusion can be achieved that enhancing cobalt content in the matrix results in rising of Curie temperature \(T_{c}\). \(Nd_{6}(Fe_{1 - x}Co_{x})_{7}B_{7}\) (\(x = 0.3\)), \(T_{c}\) reaches \(553^{\circ}C\) (Pan, Li, Xu, Ma, 2011).
Generally, by aforementioned experiment we obtained precedenless valuable information about the alloy in the in situ and dynamic observation that provided direct gist for further study of microstructure. In addition obtaining of knowledge about microstructure variation of phases around crystal interface at high tempera-ture would vail to clarify the mechanism between coercivity and microstructure.
Magnetic Properties of Nd₁₆Fe₇₇B₇: Measurement Results and Analysis
CL6 - 1 magnetic parameter measurer was use to measure magnetism of \(Nd_{16}Fe_{69}Co_{3}B_{7}\) alloy after magnetization saturation. The result measured was: remanent magnetic induction intensity is 1.28T, the intrinsic coercivity is 589.0 kA/m, the maximum magnetic energy product \((BH)_{max}=302.4\ kJ/m^{3}\). It can be seen from the result that this alloy has excellent magnetic performance. Its de-
magnetization curve is shown in Fig. 4.55.
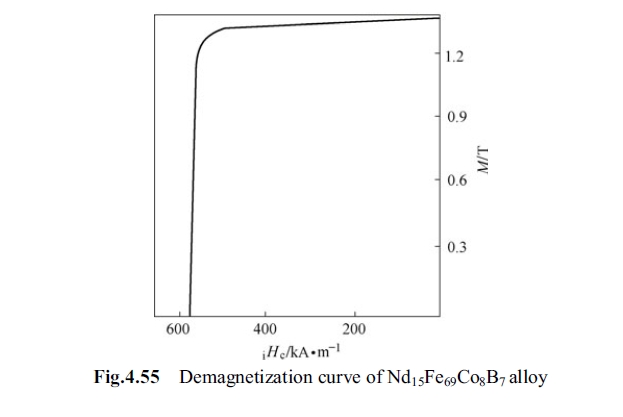
Curie Temperature Measurement of Nd₁₆Fe₇₇B₇ Permanent Magnet Alloy
Curie temperature of ternary alloy of \(Nd_{16}Fe_{77}B_{7}\) is \(312^{\circ}C\), after adding cobalt the Curie temperature is raised remarkably to be \(T_{c}=510^{\circ}C\), as shown in Fig. 4.56.
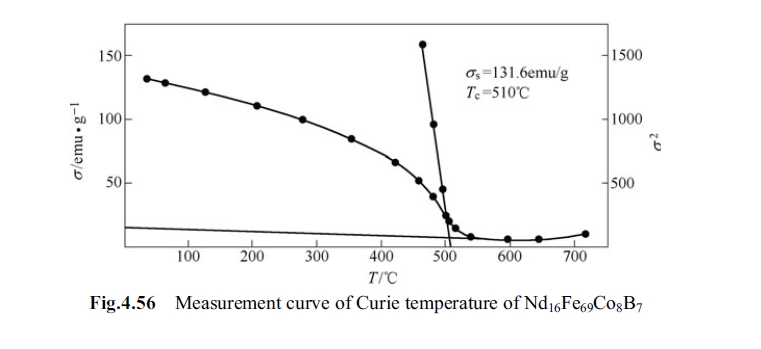
X-Ray Diffraction Phase Analysis: Lattice Constants and Cell Volume of NdFeB Alloy
X - ray diffraction pattern is shown in Fig. 4.57, lattice constant and cell volume are shown in Table 4.19.
It can be seen from Table 4.19 that \(a\), \(c\), \(V\) increased after adding cobalt to re-place part of iron.
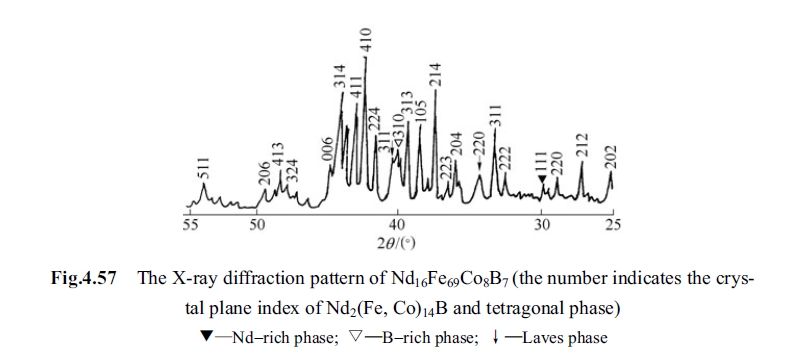
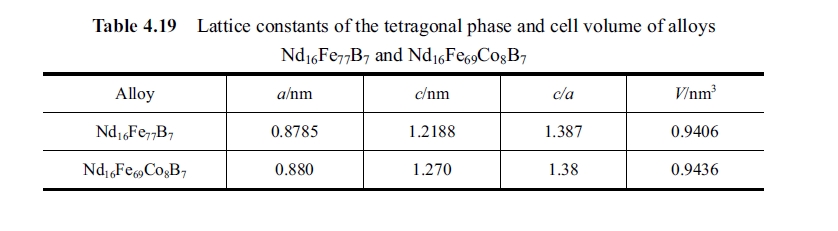
Aging Temperature and Coercivity Relationship in Nd₁₆Fe₆₉Co₈B₇ Alloys)
The intrinsic coercivity of \(Nd_{16}Fe_{69}Co_{8}B_{7}\) alloy was measured as 379 kA/m only after sintered at \(1100^{\circ}C\), and after edging at \(550^{\circ}C\) its intrinsic coercivity became 589 kA/m. The curve for relationship between aging temperature and coercivity was made at the same time, as shown as in Fig. 4.58.
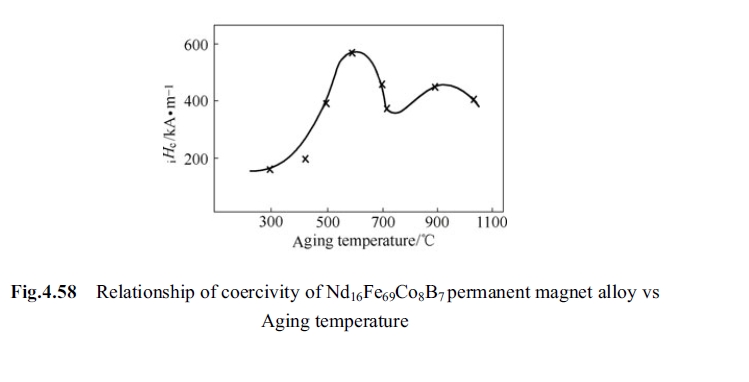
Conclusions: High-Temperature Behavior and Magnetic Performance of NdFeB Alloys
-
The in situ and dynamic observation on \(Nd_{16}Fe_{69}Co_{8}B_{7}\) alloy through HVM found that using cobalt to replace part of iron altered modality of the matrix phase of ternary NdFeB alloy, i.e., some globose \(Nd_{2}O_{3}\) impurities appeared in granule of \(Nd_{2}(Fe, Co)_{14}B\). When temperature was raised to \(400^{\circ}C\) some tiny precipitates appeared in the matrix phase, when temperature reached \(500^{\circ}C\) the precipitates grew up to be acerose and appeared largely. Nd - rich phase existed in crystal interface, which had no remarkable change in the process of raising temperature. Structure of crystalline state transferred at \(700^{\circ}C\). It can be seen from the diffraction pattern that the pattern of original multi - crystal ring became that of a non - crystalline diffusion ring and some diffraction spots at \(700^{\circ}C\) and holding for 20 min.
-
The dynamic observation found that temperature the precipitates appeared in the matrix phase precipitates was raised \(120^{\circ}C\) in comparison with ternary NdFeB alloy, i.e., the thermal stability was heightened \(120^{\circ}C\) by addition of cobalt. This result is just responding to the measuring result that Curie temperature was heightened in \(150^{\circ}C\) by addition of cobalt to replace part of iron.
-
X - ray diffraction and metallographic study result indicate that lattice constants \(a\), \(c\) were increased and crystal cell volume was augmented by adding cobalt to replace part of iron.