2.4 Analysis of Coercivity Variation and Phase Transformation in SmCo₅ Magnets
Coercivity of SmCo₅ permanent magnets presented nonlinear variation through annealing at different temperature. The outstanding specialty of this nonlinear variation is that the coercivity degraded drastically by short time annealing (heat treatment) at 700 - 750°C and afterwards the coercivity was restored by heating at 900°C, which was shown as the curve in Fig. 2.2 (Pan, Jin, 1990). The above specialties attracted great attention of scholars.
Broeder and Smegill, et al. believed that the degradation in coercivity of SmCo5 magnet by heating at 700 - 750°C in short time was related to the eutectoid decomposition; but Kumar, et al. considered that heating at 700 - 750°C in short time never could cause the eutectoid decomposition. The linear variation of the coercivity was caused by the other reasons, in fact this nonlinear variation already appeared at below 600°C but the variation was not so more remarkable as that in 700 - 750°C so that it usually be neglected. This section is mainly to introduce the peak value of coercivity of SmCo5 permanent magnet measured in annealing at below 600°C (Broeder, et al, 1972; Smegill, et al, 1973; Kamar, Das, Wettstein, 1978; Pan, Tan, Jin, Yu, 1983).
Specimen Preparation and Experimental Methods for Studying Coercivity and Phase Transformatio
All specimens were prepared by alloy melting method. The alloy was melted by arc furnace with the composition as per the proportion of metallic Sm:Co as 1:5 and among the raw materials 11% was in liquid phase with the composition of samarium 60% and cobalt 40% in mass percentage. The melted alloy was crushed roughly and then pulverized into about 5μm. The powder was oriented under a strong magnetic field and molded. Then the sample was ready for measurement after sintering.
The measurement instrument used in this experiment was magnetic parameter measurer. Measurement of the coercivity was conducted at room temperature. The experiments used JEM - 1000 HVEM to heat and dynamic observe the speci - mens. The specimen for electronic microscope was prepared as follows: sliced the sample into lamellae of 0.03mm and afterwards being ionic thinned to about 100 nm; the thinned specimen was placed in enclosed into the side inserting type heating dais of JEM - 1000 for heating and dynamic observation; the accelerating voltage was 1000 kV and electron beam was 10 μA.
Experimental Results and Discussion on Coercivity Changes in SmCo₅ Magnets
The coercivity variation curve is showed as Fig. 2.2 for specimen after annealing at different temperature. The coercivity peak at below 600°C for different specimens is showed as Fig. 2.26.
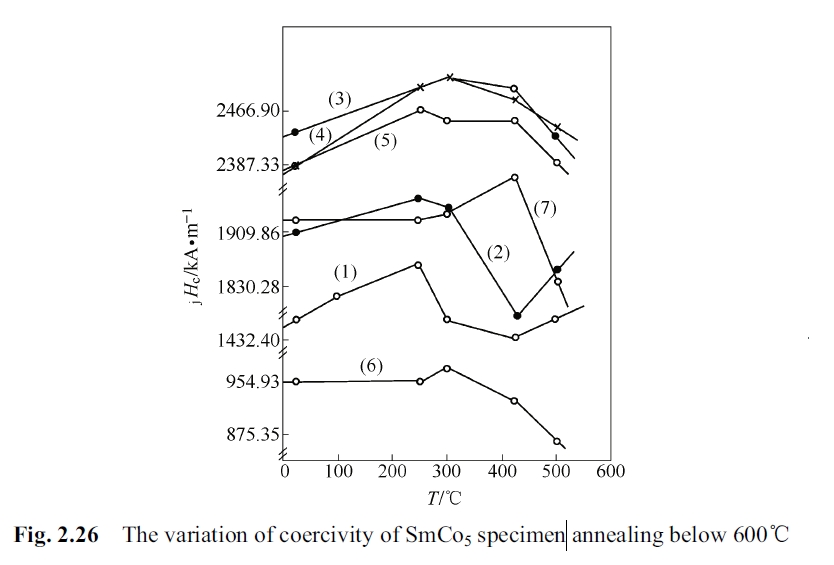
Magnetic loss is constituted with reversible loss and irreversible loss. Magnetic performance can be restored through magnetizing (magnetization). Magnetic irreversible loss can be divided into two parts, i.e., initial loss and additional loss. Initial loss is the magnetic loss caused by short term aging but additional loss is the magnetic loss caused by long term aging. In process of long term aging most of magnetic irreversible loss can be restored after re - magnetization so that only a small part of magnetic irreversible loss can not be restored.
Authors found in the experiment that the intrinsic coercivity was 2706–3024kA/m but the magnetic irreversible loss of SmCo5 specimen after long term aging at 180°C was only 1.4%–1.9%. And that 1.4% of magnetic irreversible loss is goods sample of the highest coercivity and good quadrate degree (quadrate degree) of demagnetization curve, and 1.9% magnetic irreversible loss is a specimen of the coercivity of 2706kA/m and bad ridge degree of the demagnetization curve.
All SmCo5 used in the environment above 180°C should pass this aging experiment (stabilization treatment). It can be seen from above analysis that the peak value of coercivity is related to ridgy degree in the demagnetization curve of the intrinsic coercivity of specimens.
It can be seen from Fig. 2.26 that the measured coercivity of specimens appeared peak value at below 600°C and appeared concave at 420°C. After the coercivity peak value of the magnet was found in above mentioned condition different specimens was measured as well and the result is shown in Fig. 2.26. How to explain this phenomenon? The coercivity was varies very sensitively along with variation in microtexture and structure caused by phase transformation, to investigate specialty of phase transformation and microtexture is the base to study coercivity mechanism of SmCo5 alloy. Thus using advanced microanalysis instrument enables directly observation in condition of heating under high vacuum environment and that resulted in phase growing, extending and dissolving of phase at different temperature and mutual relation and interaction between geometrical shapes study of the phase and its structure. It is necessary to understand physical hypostasis of coercivity and above mentioned experimental phenomenon.
It is known from equilibrium diagram of Sm - Co (Fig. 2.27) and relative curve of Sm - Co composition versus free energy that the SmCo5 is comparatively stable at 800°C but is metastable at below 800°C.
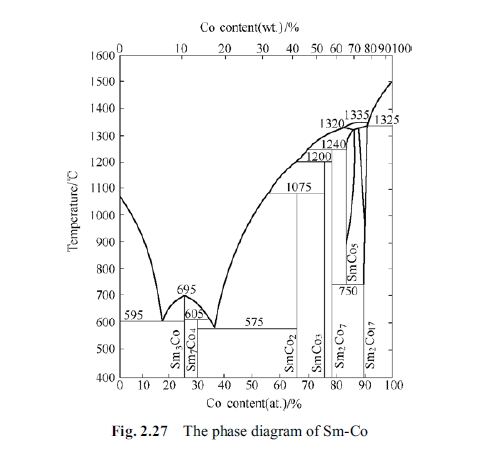
The eutectoid decomposition occurred in SmCo5 magnet at below 750°C, i.e., SmCo5 → Sm2Co7 + Sm2Co17. There is big variation for this problem as mentioned in the introduction, but the dynamic process of eutectoid decomposition in a special condition, phase growing, extending and dissolving in thermal state and geometric shape were revealed by dynamic observation experiment using 1000kV HVEM with 0.204nm resolving power in General Research Institute for Nonferrous metals (Pan, Tan, Jin, Yu, 1983). At room temperature most of areas of SmCo5 specimen does not have precipitated phase but once in a while the defect like stowage layer dislocation and very small size precipitated phase could be observed, as shown in Fig. 2.28. The specimen was samarium enriched SmCo5 sinter (including 37.66% (wt.) Sm) being sintered at 1150°C and quenched from 900°C. This type of specimen not only had defects but even also had pinhole in some small areas, as shown in Fig. 2.29. Fig. 2.29 showed photograph of magnetic domain structure of specimen at room temperature observed by Korr optic - magnetic effect (600×). It can be seen from Fig.2.29 that pattern of the magnetic domain located in middle of the pinhole.
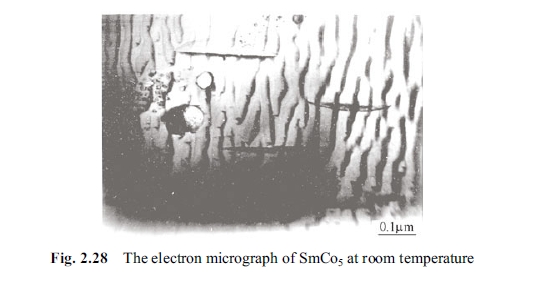
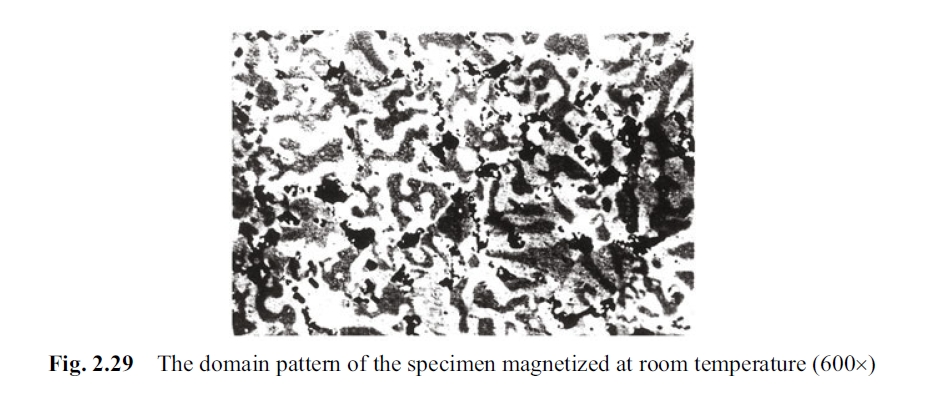
After the specimen was heated to 420°C in the 1000 kV transmission electronic microscope and held for 2 min, the specimen in the area originally without any precipitate could see clearly highly dispersed precipitate phase filled the all vision. After holding 10 min continually the precipitated phase grew to the size of teens nanometers and some precipitate phase already aggregated, as shown in Fig. 2.30 (the black precipitate was analyzed to be Sm2Co17 by electronic diffraction, R and Q in the Fig. 2.30(a) already aggregated together in the Fig. 2.30(b)). The electronic diffraction photograph, electronic diffraction pattern for selected area of precipitate phase and indexation are shown in Fig. 2.31 for SmCo5 speci -
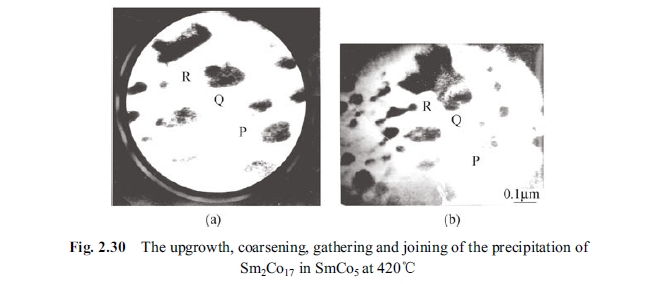
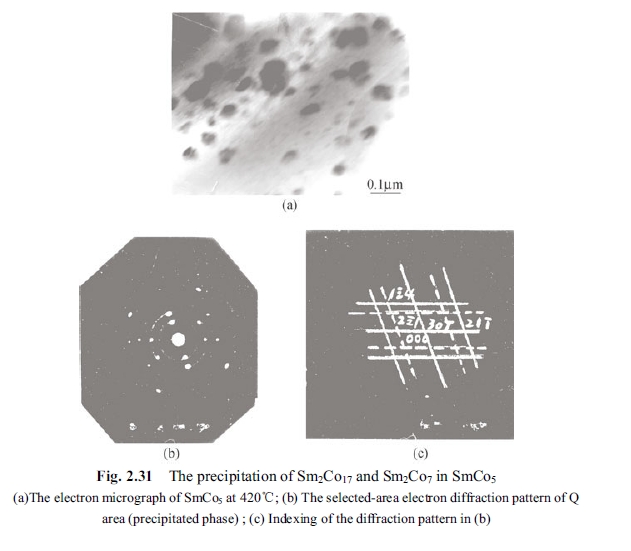
men at 420°C. The analysis of electronic diffraction of SmCo5 specimen at 420°C indicated that the precipitate phase was Sm2Co7 and Sm2Co7.
For which one of Sm2Co7 and Sm2Co17 had ever precipitated at first Fidler had made systematic investigation on interaction between dislocation in SmCo5 and the second phase. He supposed at first that the base substance had the same elasticity as that of the precipitate phase and both were isotropic when precipitate phase appeared as oblate elliptical spherical the elastic aberrance energy of a cell in the precipitate phase could be derived.
The elastic aberrance energy of Sm2Co7:
\(G_{1}^{*}=(0.043 + 0.174\ Y/Z)\)
The elastic aberrance energy of Sm2Co17:
\(G_{2}^{*}=(-0.908 +0.1736\ Y/Z)\)
The \(Z\) and \(Y\) respectively indicate the short and long radius of the oblate elliptical ball precipitate phase in the formulae. In comparison between the \(G_{1}^{*}\) and \(G_{2}^{*}\) it can be seen that \(G_{1}^{*}>G_{2}^{*}\). Thus regarding the elastic aberrance energy the precipitation of Sm2Co7 is more favorable so that Sm2Co7 phase precipitated firstly in SmCo5.
When the temperature was heightened to 500°C many precipitate phase were still in stable state. At above 600°C the imperfection in the precipitate phase could be observed obviously, which in Sm2Co17 may be caused by concentration fluctuating and short path clustering of the matrix phase. This section does not intend to treat the relation between phase precipitation and coercivity but emphases on discussion the peak value of coercivity measured at room temperature after annealing at below 600°C.
We can find without difficulties from the above mentioned observation by electronic microscope that the defect, pinholes of the specimen in thermal state due to precipitation, growing up…, and quenching of Sm2Co7 and Sm2Co17 phases are not the same as those of specimen without annealing in size and distribution. Then does what kind of the influence of the newly formed defects on impose on coercivity. The original defects disappeared thus to influence pinning of crystal boundary on the wall of magnetic domain. If the newly formed defect size is bigger than or equal to the wide of magnetic domain wall, the nucleation center will be nearby the defect and the defect will only be localized there so that this kind of defect will restrict expanding of the anti - magnetization nucleus. This kind of pinning may enhance the coercivity so that the peak value appeared in measurement. The newly formed defect when the specimen was heated again and quenched to room temperature would be different from the defect before. If this kind of defect is avail of restricting expansion of anti - magnetization nucleus then the coercivity would be enhanced further and the peak value be increased (Pan, Tian, Jin, Yu
1983; Yang, 1979). If just in opposition then the coercivity will be degraded so that become a concavity. The different specimen appeared different defects after quenching from 900°C due to technical diversity thus as the result not all of the appearance of the coercivity peak value is the same in annealing treatment. Thus annealing at a special temperature (such as just select the temperature at which m\(H_{c}\) peak value appears) is avail of enhancement of m\(H_{c}\).
Conclusions: Key Findings on Coercivity Variation and Phase Transformation
Experiments indicated that samarium enriched SmCo5 magnet sinters in all had a peak value in the variation curve of coercivity versus temperature being measured at room temperature after annealing at different temperature below 600°C. It was observed directly the matrix phase of SmCo5 eutectoid decomposed into Sm2Co7 and Sm2Co17 phases at 400 - 600°C in heating dynamic observation with 1000 kV HVEM.